Tissue Chips and Microphysiological Systems for Disease Modeling and Drug Testing
- PMID: 33525451
- PMCID: PMC7911320
- DOI: 10.3390/mi12020139
Tissue Chips and Microphysiological Systems for Disease Modeling and Drug Testing
Abstract
Tissue chips (TCs) and microphysiological systems (MPSs) that incorporate human cells are novel platforms to model disease and screen drugs and provide an alternative to traditional animal studies. This review highlights the basic definitions of TCs and MPSs, examines four major organs/tissues, identifies critical parameters for organization and function (tissue organization, blood flow, and physical stresses), reviews current microfluidic approaches to recreate tissues, and discusses current shortcomings and future directions for the development and application of these technologies. The organs emphasized are those involved in the metabolism or excretion of drugs (hepatic and renal systems) and organs sensitive to drug toxicity (cardiovascular system). This article examines the microfluidic/microfabrication approaches for each organ individually and identifies specific examples of TCs. This review will provide an excellent starting point for understanding, designing, and constructing novel TCs for possible integration within MPS.
Keywords: body-on-a-chip; microfluidics; microphysiological systems; organ-on-a-chip; tissue chips; tissue-on-a-chip.
Conflict of interest statement
The authors report no conflicts of interest.
Figures
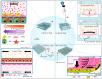
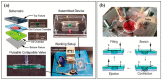
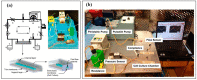
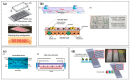
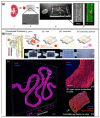
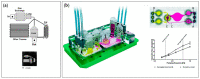
Similar articles
-
Microphysiological Systems (Tissue Chips) and their Utility for Rare Disease Research.Adv Exp Med Biol. 2017;1031:405-415. doi: 10.1007/978-3-319-67144-4_23. Adv Exp Med Biol. 2017. PMID: 29214585 Review.
-
Fitting tissue chips and microphysiological systems into the grand scheme of medicine, biology, pharmacology, and toxicology.Exp Biol Med (Maywood). 2017 Oct;242(16):1559-1572. doi: 10.1177/1535370217732765. Exp Biol Med (Maywood). 2017. PMID: 29065799 Free PMC article.
-
Engineering Microphysiological Immune System Responses on Chips.Trends Biotechnol. 2020 Aug;38(8):857-872. doi: 10.1016/j.tibtech.2020.01.003. Epub 2020 Feb 18. Trends Biotechnol. 2020. PMID: 32673588 Free PMC article. Review.
-
Organs-on-chips technologies - A guide from disease models to opportunities for drug development.Biosens Bioelectron. 2023 Jul 1;231:115271. doi: 10.1016/j.bios.2023.115271. Epub 2023 Mar 31. Biosens Bioelectron. 2023. PMID: 37060819 Review.
-
Standardizing designed and emergent quantitative features in microphysiological systems.Nat Biomed Eng. 2024 Aug;8(8):941-962. doi: 10.1038/s41551-024-01236-0. Epub 2024 Aug 26. Nat Biomed Eng. 2024. PMID: 39187664 Review.
Cited by
-
von Willebrand Factor and Angiopoietin-2 are Sensitive Biomarkers of Pulsatility in Continuous-Flow Ventricular Assist Device Patients.ASAIO J. 2023 Jun 1;69(6):569-575. doi: 10.1097/MAT.0000000000001886. Epub 2023 Mar 31. ASAIO J. 2023. PMID: 37000917 Free PMC article.
-
Tissue chips as headway model and incitement technology.Synth Syst Biotechnol. 2024 Aug 30;10(1):86-101. doi: 10.1016/j.synbio.2024.08.007. eCollection 2025. Synth Syst Biotechnol. 2024. PMID: 39286054 Free PMC article. Review.
-
Nanosafety: An Evolving Concept to Bring the Safest Possible Nanomaterials to Society and Environment.Nanomaterials (Basel). 2022 May 25;12(11):1810. doi: 10.3390/nano12111810. Nanomaterials (Basel). 2022. PMID: 35683670 Free PMC article. Review.
-
Lymph Nodes-On-Chip: Promising Immune Platforms for Pharmacological and Toxicological Applications.Front Pharmacol. 2021 Aug 16;12:711307. doi: 10.3389/fphar.2021.711307. eCollection 2021. Front Pharmacol. 2021. PMID: 34483920 Free PMC article. Review.
-
Blood-brain-barrier modeling with tissue chips for research applications in space and on Earth.Front Space Technol. 2023;4:1176943. doi: 10.3389/frspt.2023.1176943. Epub 2023 Aug 9. Front Space Technol. 2023. PMID: 38915909 Free PMC article.
References
-
- Tagle D. National Center for Advancing Translational Sciences: About Tissue Chip. [(accessed on 28 November 2020)]; Available online: https://ncats.nih.gov/tissuechip/about.
-
- Tagle D. National Center for Advancing Translational Sciences: Tissue Chip Initiatives & Projects. [(accessed on 28 November 2020)]; Available online: https://ncats.nih.gov/tissuechip/projects.
Publication types
Grants and funding
LinkOut - more resources
Full Text Sources
Other Literature Sources