An Update on Self-Amplifying mRNA Vaccine Development
- PMID: 33525396
- PMCID: PMC7911542
- DOI: 10.3390/vaccines9020097
An Update on Self-Amplifying mRNA Vaccine Development
Abstract
This review will explore the four major pillars required for design and development of an saRNA vaccine: Antigen design, vector design, non-viral delivery systems, and manufacturing (both saRNA and lipid nanoparticles (LNP)). We report on the major innovations, preclinical and clinical data reported in the last five years and will discuss future prospects.
Keywords: RNA; drug delivery; replicon; self-amplifying RNA; vaccine.
Conflict of interest statement
A.J.G. and S.I. are employees of Precision NanoSystems, Inc. and A.K.B. is a co-founder of VaxEquity and VacEquity Global Health.
Figures
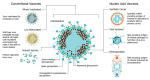
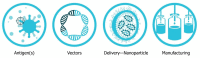
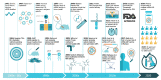
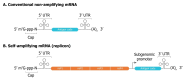
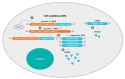
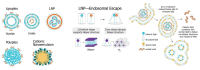
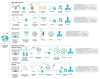
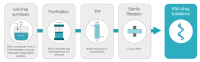
Similar articles
-
Polymeric and lipid nanoparticles for delivery of self-amplifying RNA vaccines.J Control Release. 2021 Oct 10;338:201-210. doi: 10.1016/j.jconrel.2021.08.029. Epub 2021 Aug 18. J Control Release. 2021. PMID: 34418521 Free PMC article.
-
Production, Characterization, and Assessment of Permanently Cationic and Ionizable Lipid Nanoparticles for Use in the Delivery of Self-Amplifying RNA Vaccines.Pharmaceutics. 2023 Apr 7;15(4):1173. doi: 10.3390/pharmaceutics15041173. Pharmaceutics. 2023. PMID: 37111658 Free PMC article.
-
Evaluation of a self-amplifying mRNA reporter vaccine in explant models of broiler chickens.Poult Sci. 2023 Nov;102(11):103078. doi: 10.1016/j.psj.2023.103078. Epub 2023 Aug 30. Poult Sci. 2023. PMID: 37801866 Free PMC article.
-
Current Status and Future Perspectives on MRNA Drug Manufacturing.Mol Pharm. 2022 Apr 4;19(4):1047-1058. doi: 10.1021/acs.molpharmaceut.2c00010. Epub 2022 Mar 3. Mol Pharm. 2022. PMID: 35238565 Review.
-
Self-Amplifying RNA Vaccine Candidates: Alternative Platforms for mRNA Vaccine Development.Pathogens. 2023 Jan 13;12(1):138. doi: 10.3390/pathogens12010138. Pathogens. 2023. PMID: 36678486 Free PMC article. Review.
Cited by
-
Leishmaniasis Vaccines: Applications of RNA Technology and Targeted Clinical Trial Designs.Pathogens. 2022 Oct 29;11(11):1259. doi: 10.3390/pathogens11111259. Pathogens. 2022. PMID: 36365010 Free PMC article. Review.
-
mRNA-Based Therapeutics in Cancer Treatment.Pharmaceutics. 2023 Feb 13;15(2):622. doi: 10.3390/pharmaceutics15020622. Pharmaceutics. 2023. PMID: 36839944 Free PMC article. Review.
-
mRNA melanoma vaccine revolution spurred by the COVID-19 pandemic.Front Immunol. 2023 Mar 30;14:1155728. doi: 10.3389/fimmu.2023.1155728. eCollection 2023. Front Immunol. 2023. PMID: 37063845 Free PMC article. Review.
-
Progress and prospects of mRNA-based drugs in pre-clinical and clinical applications.Signal Transduct Target Ther. 2024 Nov 14;9(1):322. doi: 10.1038/s41392-024-02002-z. Signal Transduct Target Ther. 2024. PMID: 39543114 Free PMC article. Review.
-
Self-amplifying mRNA bicistronic influenza vaccines raise cross-reactive immune responses in mice and prevent infection in ferrets.Mol Ther Methods Clin Dev. 2022 Oct 3;27:195-205. doi: 10.1016/j.omtm.2022.09.013. eCollection 2022 Dec 8. Mol Ther Methods Clin Dev. 2022. PMID: 36320414 Free PMC article.
References
Publication types
Grants and funding
LinkOut - more resources
Full Text Sources
Other Literature Sources