Molecular Modelling Guided Modulation of Molecular Shape and Charge for Design of Smart Self-Assembled Polymeric Drug Transporters
- PMID: 33499130
- PMCID: PMC7912381
- DOI: 10.3390/pharmaceutics13020141
Molecular Modelling Guided Modulation of Molecular Shape and Charge for Design of Smart Self-Assembled Polymeric Drug Transporters
Abstract
Nanomedicine employs molecular materials for prevention and treatment of disease. Recently, smart nanoparticle (NP)-based drug delivery systems were developed for the advanced transport of drug molecules. Rationally engineered organic and inorganic NP platforms hold the promise of improving drug targeting, solubility, prolonged circulation, and tissue penetration. However, despite great progress in the synthesis of NP building blocks, more interdisciplinary research is needed to understand their self-assembly and optimize their performance as smart nanocarriers. Multi-scale modeling and simulations provide a valuable ally to experiment by mapping the potential energy landscape of self-assembly, translocation, and delivery of smart drug-loaded NPs. Here, we highlight key recent advances to illustrate the concepts, methods, and applications of smart polymer-based NP drug delivery. We summarize the key design principles emerging for advanced multifunctional polymer topologies, illustrating how the unusual architecture and chemistry of dendritic polymers, self-assembling polyelectrolytes and cyclic polymers can provide exceptional drug delivery platforms. We provide a roadmap outlining the opportunities and challenges for the effective use of predictive multiscale molecular modeling techniques to accelerate the development of smart polymer-based drug delivery systems.
Keywords: cyclic polymers; dendritic polymers; molecular modeling; polyelectrolytes; self-assembly; smart drug nanocarriers.
Conflict of interest statement
The authors declare no conflict of interest.
Figures
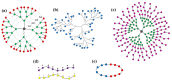
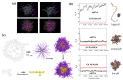
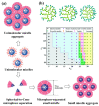
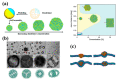

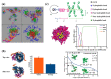
Similar articles
-
Supramolecular dendritic polymers: from synthesis to applications.Acc Chem Res. 2014 Jul 15;47(7):2006-16. doi: 10.1021/ar500057e. Epub 2014 Apr 29. Acc Chem Res. 2014. PMID: 24779892
-
Tumor-Acidity-Cleavable Maleic Acid Amide (TACMAA): A Powerful Tool for Designing Smart Nanoparticles To Overcome Delivery Barriers in Cancer Nanomedicine.Acc Chem Res. 2018 Nov 20;51(11):2848-2856. doi: 10.1021/acs.accounts.8b00195. Epub 2018 Oct 15. Acc Chem Res. 2018. PMID: 30346728 Review.
-
Macromolecular crowding: chemistry and physics meet biology (Ascona, Switzerland, 10-14 June 2012).Phys Biol. 2013 Aug;10(4):040301. doi: 10.1088/1478-3975/10/4/040301. Epub 2013 Aug 2. Phys Biol. 2013. PMID: 23912807
-
Self-assembled polymeric vesicles: Focus on polymersomes in cancer treatment.J Control Release. 2021 Feb 10;330:502-528. doi: 10.1016/j.jconrel.2020.12.027. Epub 2020 Dec 20. J Control Release. 2021. PMID: 33358973 Review.
-
Cooperative macromolecular self-assembly toward polymeric assemblies with multiple and bioactive functions.Acc Chem Res. 2014 Apr 15;47(4):1426-37. doi: 10.1021/ar5000264. Epub 2014 Apr 2. Acc Chem Res. 2014. PMID: 24694280 Review.
Cited by
-
Modeling Polyzwitterion-Based Drug Delivery Platforms: A Perspective of the Current State-of-the-Art and Beyond.ACS Eng Au. 2022 Aug 17;2(4):274-294. doi: 10.1021/acsengineeringau.2c00008. Epub 2022 May 3. ACS Eng Au. 2022. PMID: 35996394 Free PMC article. Review.
-
Form Equals Function: Influence of Coacervate Architecture on Drug Delivery Applications.ACS Biomater Sci Eng. 2024 Nov 11;10(11):6766-6789. doi: 10.1021/acsbiomaterials.4c01105. Epub 2024 Oct 18. ACS Biomater Sci Eng. 2024. PMID: 39423330 Free PMC article. Review.
-
Evaluation of Encapsulation Potential of Selected Star-Hyperbranched Polyglycidol Architectures: Predictive Molecular Dynamics Simulations and Experimental Validation.Molecules. 2023 Oct 28;28(21):7308. doi: 10.3390/molecules28217308. Molecules. 2023. PMID: 37959728 Free PMC article.
-
Polymeric Nanostructures Containing Proteins and Peptides for Pharmaceutical Applications.Polymers (Basel). 2022 Feb 16;14(4):777. doi: 10.3390/polym14040777. Polymers (Basel). 2022. PMID: 35215689 Free PMC article. Review.
References
-
- Pippa N., Demetzos S.P., Demetzos C. Polymer Self-Assembled Nanostructures as Innovative Drug Nanocarrier Platforms. [(accessed on 6 January 2021)]; Available online: https://www.eurekaselect.com/139597/article. - PubMed
Publication types
Grants and funding
LinkOut - more resources
Full Text Sources
Other Literature Sources
Miscellaneous