Single-Cell Sequencing of Brain Cell Transcriptomes and Epigenomes
- PMID: 33412093
- PMCID: PMC7808568
- DOI: 10.1016/j.neuron.2020.12.010
Single-Cell Sequencing of Brain Cell Transcriptomes and Epigenomes
Abstract
Single-cell sequencing technologies, including transcriptomic and epigenomic assays, are transforming our understanding of the cellular building blocks of neural circuits. By directly measuring multiple molecular signatures in thousands to millions of individual cells, single-cell sequencing methods can comprehensively characterize the diversity of brain cell types. These measurements uncover gene regulatory mechanisms that shape cellular identity and provide insight into developmental and evolutionary relationships between brain cell populations. Single-cell sequencing data can aid the design of tools for targeted functional studies of brain circuit components, linking molecular signatures with anatomy, connectivity, morphology, and physiology. Here, we discuss the fundamental principles of single-cell transcriptome and epigenome sequencing, integrative computational analysis of the data, and key applications in neuroscience.
Keywords: ATAC-seq; DNA methylation; cell state; cell type; epigenome; multi-omics; open chromatin; single-cell sequencing; spatial transcriptomics; transcriptome.
Copyright © 2020 Elsevier Inc. All rights reserved.
Conflict of interest statement
Figures
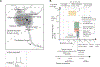
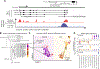
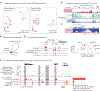
Similar articles
-
EpiScanpy: integrated single-cell epigenomic analysis.Nat Commun. 2021 Sep 1;12(1):5228. doi: 10.1038/s41467-021-25131-3. Nat Commun. 2021. PMID: 34471111 Free PMC article.
-
Single-Cell Sequencing Methodologies: From Transcriptome to Multi-Dimensional Measurement.Small Methods. 2021 Jun;5(6):e2100111. doi: 10.1002/smtd.202100111. Epub 2021 Apr 17. Small Methods. 2021. PMID: 34927917 Review.
-
Single-Cell Analysis of the Transcriptome and Epigenome.Methods Mol Biol. 2022;2399:21-60. doi: 10.1007/978-1-0716-1831-8_3. Methods Mol Biol. 2022. PMID: 35604552 Free PMC article.
-
Simultaneous Profiling of mRNA Transcriptome and DNA Methylome from a Single Cell.Methods Mol Biol. 2019;1979:363-377. doi: 10.1007/978-1-4939-9240-9_21. Methods Mol Biol. 2019. PMID: 31028648
-
Deciphering Brain Complexity Using Single-cell Sequencing.Genomics Proteomics Bioinformatics. 2019 Aug;17(4):344-366. doi: 10.1016/j.gpb.2018.07.007. Epub 2019 Oct 3. Genomics Proteomics Bioinformatics. 2019. PMID: 31586689 Free PMC article. Review.
Cited by
-
Human sensory-like neuron cultivation-An optimized protocol.Front Neurosci. 2024 Oct 3;18:1429694. doi: 10.3389/fnins.2024.1429694. eCollection 2024. Front Neurosci. 2024. PMID: 39420988 Free PMC article.
-
Advancements in Single-Cell RNA Sequencing and Spatial Transcriptomics for Central Nervous System Disease.Cell Mol Neurobiol. 2024 Oct 10;44(1):65. doi: 10.1007/s10571-024-01499-w. Cell Mol Neurobiol. 2024. PMID: 39387975 Free PMC article. Review.
-
Innovative aspects and applications of single cell technology for different diseases.Am J Cancer Res. 2024 Aug 25;14(8):4028-4048. doi: 10.62347/VUFU1836. eCollection 2024. Am J Cancer Res. 2024. PMID: 39267684 Free PMC article. Review.
-
Single-cell sequencing technology in diabetic wound healing: New insights into the progenitors-based repair strategies.World J Stem Cells. 2024 May 26;16(5):462-466. doi: 10.4252/wjsc.v16.i5.462. World J Stem Cells. 2024. PMID: 38817326 Free PMC article.
-
Understanding glioblastoma at the single-cell level: Recent advances and future challenges.PLoS Biol. 2024 May 30;22(5):e3002640. doi: 10.1371/journal.pbio.3002640. eCollection 2024 May. PLoS Biol. 2024. PMID: 38814900 Free PMC article. Review.
References
-
- Arendt D, Musser JM, Baker CVH, Bergman A, Cepko C, Erwin DH, Pavlicev M, Schlosser G, Widder S, Laubichler MD, et al. (2016). The origin and evolution of cell types. Nat. Rev. Genet 17, 744–757. - PubMed
-
- Azevedo FAC, Carvalho LRB, Grinberg LT, Farfel JM, Ferretti REL, Leite REP, Jacob Filho W, Lent R, and Herculano-Houzel S (2009). Equal numbers of neuronal and nonneuronal cells make the human brain an isometrically scaled-up primate brain. J. Comp. Neurol 513, 532–541. - PubMed
-
- Bakken TE, Jorstad NL, Hu Q, Lake BB, and Tian W (2020). Evolution of cellular diversity in primary motor cortex of human, marmoset monkey, and mouse. bioRxiv.
Publication types
MeSH terms
Grants and funding
LinkOut - more resources
Full Text Sources
Other Literature Sources