Xenopus leads the way: Frogs as a pioneering model to understand the human brain
- PMID: 33369095
- PMCID: PMC8130472
- DOI: 10.1002/dvg.23405
Xenopus leads the way: Frogs as a pioneering model to understand the human brain
Abstract
From its long history in the field of embryology to its recent advances in genetics, Xenopus has been an indispensable model for understanding the human brain. Foundational studies that gave us our first insights into major embryonic patterning events serve as a crucial backdrop for newer avenues of investigation into organogenesis and organ function. The vast array of tools available in Xenopus laevis and Xenopus tropicalis allows interrogation of developmental phenomena at all levels, from the molecular to the behavioral, and the application of CRISPR technology has enabled the investigation of human disorder risk genes in a higher-throughput manner. As the only major tetrapod model in which all developmental stages are easily manipulated and observed, frogs provide the unique opportunity to study organ development from the earliest stages. All of these features make Xenopus a premier model for studying the development of the brain, a notoriously complex process that demands an understanding of all stages from fertilization to organogenesis and beyond. Importantly, core processes of brain development are conserved between Xenopus and human, underlining the advantages of this model. This review begins by summarizing discoveries made in amphibians that form the cornerstones of vertebrate neurodevelopmental biology and goes on to discuss recent advances that have catapulted our understanding of brain development in Xenopus and in relation to human development and disease. As we engage in a new era of patient-driven gene discovery, Xenopus offers exceptional potential to uncover conserved biology underlying human brain disorders and move towards rational drug design.
Keywords: amphibian; birth defects; genetics; neural; organogenesis.
© 2020 Wiley Periodicals LLC.
Conflict of interest statement
Figures
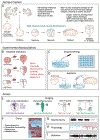
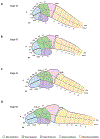
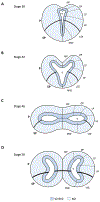
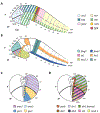
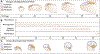
Similar articles
-
Modeling endoderm development and disease in Xenopus.Curr Top Dev Biol. 2021;145:61-90. doi: 10.1016/bs.ctdb.2021.01.001. Epub 2021 Mar 3. Curr Top Dev Biol. 2021. PMID: 34074536 Review.
-
Expanding the genetic toolkit in Xenopus: Approaches and opportunities for human disease modeling.Dev Biol. 2017 Jun 15;426(2):325-335. doi: 10.1016/j.ydbio.2016.04.009. Epub 2016 Apr 22. Dev Biol. 2017. PMID: 27109192 Free PMC article. Review.
-
The African clawed frog Xenopus laevis: A model organism to study regeneration of the central nervous system.Neurosci Lett. 2017 Jun 23;652:82-93. doi: 10.1016/j.neulet.2016.09.054. Epub 2016 Sep 29. Neurosci Lett. 2017. PMID: 27693567 Review.
-
Xenopus: Experimental Access to Cardiovascular Development, Regeneration Discovery, and Cardiovascular Heart-Defect Modeling.Cold Spring Harb Perspect Biol. 2020 Jun 1;12(6):a037200. doi: 10.1101/cshperspect.a037200. Cold Spring Harb Perspect Biol. 2020. PMID: 31767648 Free PMC article. Review.
-
Role of Thyroid Hormone Receptor in Amphibian Development.Methods Mol Biol. 2018;1801:247-263. doi: 10.1007/978-1-4939-7902-8_20. Methods Mol Biol. 2018. PMID: 29892830 Free PMC article.
Cited by
-
Production and characterization of monoclonal antibodies to Xenopus proteins.Development. 2023 Feb 15;150(4):dev201309. doi: 10.1242/dev.201309. Epub 2023 Feb 15. Development. 2023. PMID: 36789951 Free PMC article.
-
A Focal Impact Model of Traumatic Brain Injury in Xenopus Tadpoles Reveals Behavioral Alterations, Neuroinflammation, and an Astroglial Response.Int J Mol Sci. 2022 Jul 8;23(14):7578. doi: 10.3390/ijms23147578. Int J Mol Sci. 2022. PMID: 35886924 Free PMC article.
-
Modelling human genetic disorders in Xenopus tropicalis.Dis Model Mech. 2024 May 1;17(5):dmm050754. doi: 10.1242/dmm.050754. Epub 2024 Jun 4. Dis Model Mech. 2024. PMID: 38832520 Free PMC article. Review.
-
SMC5 Plays Independent Roles in Congenital Heart Disease and Neurodevelopmental Disability.Int J Mol Sci. 2023 Dec 28;25(1):430. doi: 10.3390/ijms25010430. Int J Mol Sci. 2023. PMID: 38203602 Free PMC article.
-
Genomics, convergent neuroscience and progress in understanding autism spectrum disorder.Nat Rev Neurosci. 2022 Jun;23(6):323-341. doi: 10.1038/s41583-022-00576-7. Epub 2022 Apr 19. Nat Rev Neurosci. 2022. PMID: 35440779 Free PMC article. Review.
References
-
- Ablondi Eileen F., Paudel Sudip, Sehdev Morgan, Marken John P., Halleran Andrew D., Rahman Atiqur, Kemper Peter, and Saha Margaret S.. 2020. “Fluorescent Calcium Imaging and Subsequent In Situ Hybridization for Neuronal Precursor Characterization in Xenopus Laevis.” Journal of Visualized Experiments: JoVE, no. 156 (February), 10.3791/60726. - DOI - PubMed
-
- Arendt Detlev, Musser Jacob M., Baker Clare V. H., Bergman Aviv, Cepko Connie, Erwin Douglas H., Pavlicev Mihaela, et al. 2016. “The Origin and Evolution of Cell Types.” Nature Reviews. Genetics 17 (12): 744–57. - PubMed
Publication types
MeSH terms
Grants and funding
LinkOut - more resources
Full Text Sources
Research Materials