Transcript assembly improves expression quantification of transposable elements in single-cell RNA-seq data
- PMID: 33355230
- PMCID: PMC7849386
- DOI: 10.1101/gr.265173.120
Transcript assembly improves expression quantification of transposable elements in single-cell RNA-seq data
Abstract
Transposable elements (TEs) are an integral part of the host transcriptome. TE-containing noncoding RNAs (ncRNAs) show considerable tissue specificity and play important roles during development, including stem cell maintenance and cell differentiation. Recent advances in single-cell RNA-seq (scRNA-seq) revolutionized cell type-specific gene expression analysis. However, effective scRNA-seq quantification tools tailored for TEs are lacking, limiting our ability to dissect TE expression dynamics at single-cell resolution. To address this issue, we established a TE expression quantification pipeline that is compatible with scRNA-seq data generated across multiple technology platforms. We constructed TE-containing ncRNA references using bulk RNA-seq data and showed that quantifying TE expression at the transcript level effectively reduces noise. As proof of principle, we applied this strategy to mouse embryonic stem cells and successfully captured the expression profile of endogenous retroviruses in single cells. We further expanded our analysis to scRNA-seq data from early stages of mouse embryogenesis. Our results illustrated the dynamic TE expression at preimplantation stages and revealed 146 TE-containing ncRNA transcripts with substantial tissue specificity during gastrulation and early organogenesis.
© 2021 Shao and Wang; Published by Cold Spring Harbor Laboratory Press.
Figures
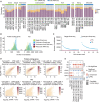
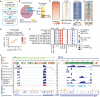
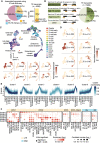
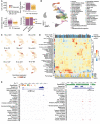
Similar articles
-
SoloTE for improved analysis of transposable elements in single-cell RNA-Seq data using locus-specific expression.Commun Biol. 2022 Oct 6;5(1):1063. doi: 10.1038/s42003-022-04020-5. Commun Biol. 2022. PMID: 36202992 Free PMC article.
-
Identifying transposable element expression dynamics and heterogeneity during development at the single-cell level with a processing pipeline scTE.Nat Commun. 2021 Mar 5;12(1):1456. doi: 10.1038/s41467-021-21808-x. Nat Commun. 2021. PMID: 33674594 Free PMC article.
-
RepExpress: A Novel Pipeline for the Quantification and Characterization of Transposable Element Expression from RNA-seq Data.Curr Protoc. 2021 Aug;1(8):e206. doi: 10.1002/cpz1.206. Curr Protoc. 2021. PMID: 34387946
-
More than causing (epi)genomic instability: emerging physiological implications of transposable element modulation.J Biomed Sci. 2021 Aug 7;28(1):58. doi: 10.1186/s12929-021-00754-2. J Biomed Sci. 2021. PMID: 34364371 Free PMC article. Review.
-
The intertwining of transposable elements and non-coding RNAs.Int J Mol Sci. 2013 Jun 26;14(7):13307-28. doi: 10.3390/ijms140713307. Int J Mol Sci. 2013. PMID: 23803660 Free PMC article. Review.
Cited by
-
Towards targeting transposable elements for cancer therapy.Nat Rev Cancer. 2024 Feb;24(2):123-140. doi: 10.1038/s41568-023-00653-8. Epub 2024 Jan 16. Nat Rev Cancer. 2024. PMID: 38228901 Review.
-
Zebrafish transposable elements show extensive diversification in age, genomic distribution, and developmental expression.Genome Res. 2022 Jul;32(7):1408-1423. doi: 10.1101/gr.275655.121. Epub 2022 Jan 5. Genome Res. 2022. PMID: 34987056 Free PMC article.
-
SoloTE for improved analysis of transposable elements in single-cell RNA-Seq data using locus-specific expression.Commun Biol. 2022 Oct 6;5(1):1063. doi: 10.1038/s42003-022-04020-5. Commun Biol. 2022. PMID: 36202992 Free PMC article.
-
Roles of transposable elements in the regulation of mammalian transcription.Nat Rev Mol Cell Biol. 2022 Jul;23(7):481-497. doi: 10.1038/s41580-022-00457-y. Epub 2022 Feb 28. Nat Rev Mol Cell Biol. 2022. PMID: 35228718 Free PMC article. Review.
-
Bioinformatics Approaches for Determining the Functional Impact of Repetitive Elements on Non-coding RNAs.Methods Mol Biol. 2022;2509:315-340. doi: 10.1007/978-1-0716-2380-0_19. Methods Mol Biol. 2022. PMID: 35796972
References
-
- Attig J, Young GR, Hosie L, Perkins D, Encheva-Yokoya V, Stoye JP, Snijders AP, Ternette N, Kassiotis G. 2019. LTR retroelement expansion of the human cancer transcriptome and immunopeptidome revealed by de novo transcript assembly. Genome Res 29: 1578–1590. 10.1101/gr.248922.119 - DOI - PMC - PubMed
-
- Benadiba C, Magnani D, Niquille M, Morlé L, Valloton D, Nawabi H, Ait-Lounis A, Otsmane B, Reith W, Theil T, et al. 2012. The ciliogenic transcription factor RFX3 regulates early midline distribution of guidepost neurons required for corpus callosum development. PLoS Genet 8: e1002606 10.1371/journal.pgen.1002606 - DOI - PMC - PubMed
-
- Bendall ML, de Mulder M, Iñiguez LP, Lecanda-Sánchez A, Pérez-Losada M, Ostrowski MA, Jones RB, Mulder LCF, Reyes-Terán G, Crandall KA, et al. 2019. Telescope: characterization of the retrotranscriptome by accurate estimation of transposable element expression. PLoS Comput Biol 15: e1006453 10.1371/journal.pcbi.1006453 - DOI - PMC - PubMed
Publication types
MeSH terms
Substances
Grants and funding
LinkOut - more resources
Full Text Sources
Research Materials