The SARS-CoV-2 RNA-protein interactome in infected human cells
- PMID: 33349665
- PMCID: PMC7906908
- DOI: 10.1038/s41564-020-00846-z
The SARS-CoV-2 RNA-protein interactome in infected human cells
Abstract
Characterizing the interactions that SARS-CoV-2 viral RNAs make with host cell proteins during infection can improve our understanding of viral RNA functions and the host innate immune response. Using RNA antisense purification and mass spectrometry, we identified up to 104 human proteins that directly and specifically bind to SARS-CoV-2 RNAs in infected human cells. We integrated the SARS-CoV-2 RNA interactome with changes in proteome abundance induced by viral infection and linked interactome proteins to cellular pathways relevant to SARS-CoV-2 infections. We demonstrated by genetic perturbation that cellular nucleic acid-binding protein (CNBP) and La-related protein 1 (LARP1), two of the most strongly enriched viral RNA binders, restrict SARS-CoV-2 replication in infected cells and provide a global map of their direct RNA contact sites. Pharmacological inhibition of three other RNA interactome members, PPIA, ATP1A1, and the ARP2/3 complex, reduced viral replication in two human cell lines. The identification of host dependency factors and defence strategies as presented in this work will improve the design of targeted therapeutics against SARS-CoV-2.
Conflict of interest statement
The authors declare no competing interests.
Figures
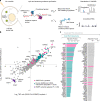
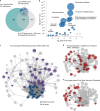
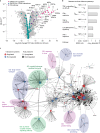
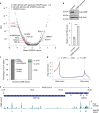
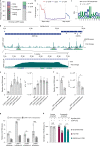
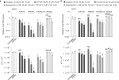
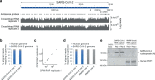
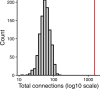
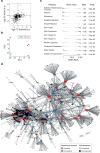
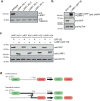
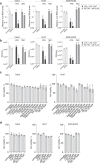
Similar articles
-
The SARS-CoV-2 RNA interactome.Mol Cell. 2021 Jul 1;81(13):2838-2850.e6. doi: 10.1016/j.molcel.2021.04.022. Epub 2021 Apr 27. Mol Cell. 2021. PMID: 33989516 Free PMC article.
-
Proteomic analysis identifies the RNA helicase DDX3X as a host target against SARS-CoV-2 infection.Antiviral Res. 2021 Jun;190:105064. doi: 10.1016/j.antiviral.2021.105064. Epub 2021 Mar 26. Antiviral Res. 2021. PMID: 33781803 Free PMC article.
-
High-sensitivity profiling of SARS-CoV-2 noncoding region-host protein interactome reveals the potential regulatory role of negative-sense viral RNA.mSystems. 2023 Aug 31;8(4):e0013523. doi: 10.1128/msystems.00135-23. Epub 2023 Jun 14. mSystems. 2023. PMID: 37314180 Free PMC article.
-
Mapping the SARS-CoV-2-Host Protein-Protein Interactome by Affinity Purification Mass Spectrometry and Proximity-Dependent Biotin Labeling: A Rational and Straightforward Route to Discover Host-Directed Anti-SARS-CoV-2 Therapeutics.Int J Mol Sci. 2021 Jan 7;22(2):532. doi: 10.3390/ijms22020532. Int J Mol Sci. 2021. PMID: 33430309 Free PMC article. Review.
-
Cellular host factors for SARS-CoV-2 infection.Nat Microbiol. 2021 Oct;6(10):1219-1232. doi: 10.1038/s41564-021-00958-0. Epub 2021 Sep 1. Nat Microbiol. 2021. PMID: 34471255 Review.
Cited by
-
Coronavirus takeover of host cell translation and intracellular antiviral response: a molecular perspective.EMBO J. 2024 Jan;43(2):151-167. doi: 10.1038/s44318-023-00019-8. Epub 2024 Jan 10. EMBO J. 2024. PMID: 38200146 Free PMC article. Review.
-
A genome-wide arrayed CRISPR screen identifies PLSCR1 as an intrinsic barrier to SARS-CoV-2 entry that recent virus variants have evolved to resist.PLoS Biol. 2024 Sep 24;22(9):e3002767. doi: 10.1371/journal.pbio.3002767. eCollection 2024 Sep. PLoS Biol. 2024. PMID: 39316623 Free PMC article.
-
SARS-CoV-2 omicron BA.5 and XBB variants have increased neurotropic potential over BA.1 in K18-hACE2 mice and human brain organoids.Front Microbiol. 2023 Nov 23;14:1320856. doi: 10.3389/fmicb.2023.1320856. eCollection 2023. Front Microbiol. 2023. PMID: 38075874 Free PMC article.
-
Discovery and functional interrogation of SARS-CoV-2 RNA-host protein interactions.Cell. 2021 Apr 29;184(9):2394-2411.e16. doi: 10.1016/j.cell.2021.03.012. Epub 2021 Mar 11. Cell. 2021. PMID: 33743211 Free PMC article.
-
COVID-19 Salivary Protein Profile: Unravelling Molecular Aspects of SARS-CoV-2 Infection.J Clin Med. 2022 Sep 22;11(19):5571. doi: 10.3390/jcm11195571. J Clin Med. 2022. PMID: 36233441 Free PMC article.
References
Publication types
MeSH terms
Substances
Grants and funding
LinkOut - more resources
Full Text Sources
Medical
Molecular Biology Databases
Research Materials
Miscellaneous