Ferroptosis drives photoreceptor degeneration in mice with defects in all-trans-retinal clearance
- PMID: 33334878
- PMCID: PMC7948481
- DOI: 10.1074/jbc.RA120.015779
Ferroptosis drives photoreceptor degeneration in mice with defects in all-trans-retinal clearance
Abstract
The death of photoreceptor cells in dry age-related macular degeneration (AMD) and autosomal recessive Stargardt disease (STGD1) is closely associated with disruption in all-trans-retinal (atRAL) clearance in neural retina. In this study, we reveal that the overload of atRAL leads to photoreceptor degeneration through activating ferroptosis, a nonapoptotic form of cell death. Ferroptosis of photoreceptor cells induced by atRAL resulted from increased ferrous ion (Fe2+), elevated ACSL4 expression, system Xc- inhibition, and mitochondrial destruction. Fe2+ overload, tripeptide glutathione (GSH) depletion, and damaged mitochondria in photoreceptor cells exposed to atRAL provoked reactive oxygen species (ROS) production, which, together with ACSL4 activation, promoted lipid peroxidation and thereby evoked ferroptotic cell death. Moreover, exposure of photoreceptor cells to atRAL activated COX2, a well-accepted biomarker for ferroptosis onset. In addition to GSH supplement, inhibiting either Fe2+ by deferoxamine mesylate salt (DFO) or lipid peroxidation with ferrostatin-1 (Fer-1) protected photoreceptor cells from ferroptosis caused by atRAL. Abca4-/-Rdh8-/- mice exhibiting defects in atRAL clearance is an animal model for dry AMD and STGD1. We observed that ferroptosis was indeed present in neural retina of Abca4-/-Rdh8-/- mice after light exposure. More importantly, photoreceptor atrophy and ferroptosis in light-exposed Abca4-/-Rdh8-/- mice were effectively alleviated by intraperitoneally injected Fer-1, a selective inhibitor of ferroptosis. Our study suggests that ferroptosis is one of the important pathways of photoreceptor cell death in retinopathies arising from excess atRAL accumulation and should be pursued as a novel target for protection against dry AMD and STGD1.
Keywords: Stargardt disease; all-trans-retinal; cell death; ferroptosis; iron metabolism; lipid peroxidation; macular degeneration; oxidative stress; photoreceptor.
Copyright © 2020 The Authors. Published by Elsevier Inc. All rights reserved.
Conflict of interest statement
Conflicts of interest The authors declare that they have no competing conflicts of interest with the contents of this article.
Figures
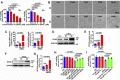
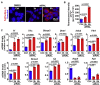
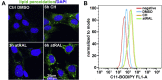
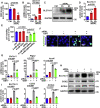
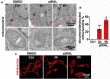
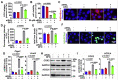
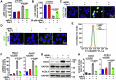
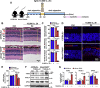
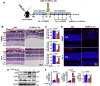
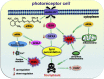
Similar articles
-
Induction of ferroptosis by HO-1 contributes to retinal degeneration in mice with defective clearance of all-trans-retinal.Free Radic Biol Med. 2023 Jan;194:245-254. doi: 10.1016/j.freeradbiomed.2022.12.008. Epub 2022 Dec 9. Free Radic Biol Med. 2023. PMID: 36509314
-
Activation of JNK signaling promotes all-trans-retinal-induced photoreceptor apoptosis in mice.J Biol Chem. 2020 May 15;295(20):6958-6971. doi: 10.1074/jbc.RA120.013189. Epub 2020 Apr 7. J Biol Chem. 2020. PMID: 32265302 Free PMC article.
-
eIF2α incites photoreceptor cell and retina damage by all-trans-retinal.J Biol Chem. 2023 May;299(5):104686. doi: 10.1016/j.jbc.2023.104686. Epub 2023 Apr 7. J Biol Chem. 2023. PMID: 37031820 Free PMC article.
-
Iron Accumulation and Lipid Peroxidation in the Aging Retina: Implication of Ferroptosis in Age-Related Macular Degeneration.Aging Dis. 2021 Apr 1;12(2):529-551. doi: 10.14336/AD.2020.0912. eCollection 2021 Apr. Aging Dis. 2021. PMID: 33815881 Free PMC article. Review.
-
Ferroptosis: process and function.Cell Death Differ. 2016 Mar;23(3):369-79. doi: 10.1038/cdd.2015.158. Epub 2016 Jan 22. Cell Death Differ. 2016. PMID: 26794443 Free PMC article. Review.
Cited by
-
Astaxanthin activated the SLC7A11/GPX4 pathway to inhibit ferroptosis and enhance autophagy, ameliorating dry eye disease.Front Pharmacol. 2024 Aug 19;15:1407659. doi: 10.3389/fphar.2024.1407659. eCollection 2024. Front Pharmacol. 2024. PMID: 39224780 Free PMC article.
-
Ferrostatin-1-loaded liposome for treatment of corneal alkali burn via targeting ferroptosis.Bioeng Transl Med. 2021 Dec 8;7(2):e10276. doi: 10.1002/btm2.10276. eCollection 2022 May. Bioeng Transl Med. 2021. PMID: 35600640 Free PMC article.
-
Flow cytometric method for the detection and quantification of retinal cell death and oxidative stress.Exp Eye Res. 2023 Aug;233:109563. doi: 10.1016/j.exer.2023.109563. Epub 2023 Jun 29. Exp Eye Res. 2023. PMID: 37393050 Free PMC article.
-
Research progress of iron metabolism in retinal diseases.Adv Ophthalmol Pract Res. 2023 Feb 28;3(2):93-100. doi: 10.1016/j.aopr.2023.02.001. eCollection 2023 May-Jun. Adv Ophthalmol Pract Res. 2023. PMID: 37846377 Free PMC article. Review.
-
Effects of Alkalinity Stress on Amino Acid Metabolism Profiles and Oxidative-Stress-Mediated Apoptosis/Ferroptosis in Hybrid Sturgeon (Huso dauricus ♀ × Acipenser schrenckii ♂) Livers.Int J Mol Sci. 2024 Sep 27;25(19):10456. doi: 10.3390/ijms251910456. Int J Mol Sci. 2024. PMID: 39408786 Free PMC article.
References
-
- Palczewski K., Kumasaka T., Hori T., Behnke C.A., Motoshima H., Fox B.A., Le Trong I., Teller D.C., Okada T., Stenkamp R.E., Yamamoto M., Miyano M. Crystal structure of rhodopsin: a G protein-coupled receptor. Science. 2000;289:739–745. - PubMed
-
- Rando R.R. Polyenes and vision. Chem. Biol. 1996;3:255–262. - PubMed
-
- Liu X., Chen J., Liu Z., Li J., Yao K., Wu Y. Potential therapeutic agents against retinal diseases caused by aberrant metabolism of retinoids. Invest. Ophthalmol. Vis. Sci. 2016;57:1017–1030. - PubMed
Publication types
MeSH terms
Substances
LinkOut - more resources
Full Text Sources
Other Literature Sources
Medical
Research Materials