Thermoresponsive Polypeptoids
- PMID: 33322804
- PMCID: PMC7763442
- DOI: 10.3390/polym12122973
Thermoresponsive Polypeptoids
Abstract
Stimuli-responsive polymers have been widely studied in many applications such as biomedicine, nanotechnology, and catalysis. Temperature is one of the most commonly used external triggers, which can be highly controlled with excellent reversibility. Thermoresponsive polymers exhibiting a reversible phase transition in a controlled manner to temperature are a promising class of smart polymers that have been widely studied. The phase transition behavior can be tuned by polymer architectures, chain-end, and various functional groups. Particularly, thermoresponsive polypeptoid is a type of promising material that has drawn growing interest because of its excellent biocompatibility, biodegradability, and bioactivity. This paper summarizes the recent advances of thermoresponsive polypeptoids, including the synthetic methods and functional groups as well as their applications.
Keywords: polypeptoids; post-polymerization modification; ring-opening polymerization (ROP); solid-phase synthesis; thermoresponsive.
Conflict of interest statement
The authors declare no conflict of interest.
Figures
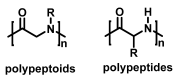
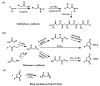
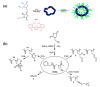
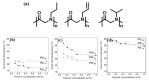

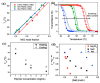
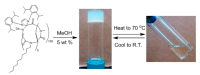
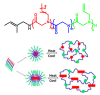
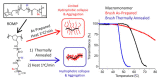
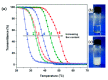
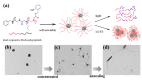

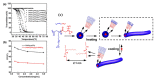
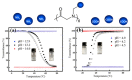
Similar articles
-
Recent Advances in Thermoresponsive OEGylated Poly(amino acid)s.Polymers (Basel). 2021 May 31;13(11):1813. doi: 10.3390/polym13111813. Polymers (Basel). 2021. PMID: 34072769 Free PMC article. Review.
-
Charge-Determined LCST/UCST Behavior in Ionic Polypeptoids.Biomacromolecules. 2018 Jun 11;19(6):2109-2116. doi: 10.1021/acs.biomac.8b00240. Epub 2018 Apr 17. Biomacromolecules. 2018. PMID: 29664626
-
Tunable LCST/UCST-Type Polypeptoids and Their Structure-Property Relationship.Biomacromolecules. 2020 Dec 14;21(12):4980-4988. doi: 10.1021/acs.biomac.0c01177. Epub 2020 Oct 14. Biomacromolecules. 2020. PMID: 33307699
-
Stimulus-Responsive Polymers Based on Polypeptoid Skeletons.Polymers (Basel). 2021 Jun 24;13(13):2089. doi: 10.3390/polym13132089. Polymers (Basel). 2021. PMID: 34202869 Free PMC article. Review.
-
Dual-responsive pegylated polypeptoids with tunable cloud point temperatures.Biopolymers. 2019 Apr;110(4):e23243. doi: 10.1002/bip.23243. Epub 2018 Dec 8. Biopolymers. 2019. PMID: 30537092
Cited by
-
Photothermally responsive theranostic nanocomposites for near-infrared light triggered drug release and enhanced synergism of photothermo-chemotherapy for gastric cancer.Bioeng Transl Med. 2022 Jul 12;8(1):e10368. doi: 10.1002/btm2.10368. eCollection 2023 Jan. Bioeng Transl Med. 2022. PMID: 36684111 Free PMC article.
-
Adamantane Functionalized Poly(2-oxazoline)s with Broadly Tunable LCST-Behavior by Molecular Recognition.Polymers (Basel). 2021 Jan 26;13(3):374. doi: 10.3390/polym13030374. Polymers (Basel). 2021. PMID: 33530443 Free PMC article.
-
Cyclodextrin-Based Polymeric Drug Delivery Systems for Cancer Therapy.Polymers (Basel). 2023 Mar 11;15(6):1400. doi: 10.3390/polym15061400. Polymers (Basel). 2023. PMID: 36987181 Free PMC article. Review.
-
A Field Guide to Optimizing Peptoid Synthesis.ACS Polym Au. 2022 Dec 14;2(6):417-429. doi: 10.1021/acspolymersau.2c00036. Epub 2022 Sep 15. ACS Polym Au. 2022. PMID: 36536890 Free PMC article. Review.
-
Chitosan-Based Biocompatible Copolymers for Thermoresponsive Drug Delivery Systems: On the Development of a Standardization System.Pharmaceutics. 2021 Nov 5;13(11):1876. doi: 10.3390/pharmaceutics13111876. Pharmaceutics. 2021. PMID: 34834291 Free PMC article. Review.
References
-
- Pasparakis G., Tsitsilianis C. LCST polymers: Thermoresponsive nanostructured assemblies towards bioapplications. Polymer. 2020;211:123146. doi: 10.1016/j.polymer.2020.123146. - DOI
Publication types
Grants and funding
LinkOut - more resources
Full Text Sources