Deep imaging in the brainstem reveals functional heterogeneity in V2a neurons controlling locomotion
- PMID: 33277252
- PMCID: PMC7821901
- DOI: 10.1126/sciadv.abc6309
Deep imaging in the brainstem reveals functional heterogeneity in V2a neurons controlling locomotion
Abstract
V2a neurons are a genetically defined cell class that forms a major excitatory descending pathway from the brainstem reticular formation to the spinal cord. Their activation has been linked to the termination of locomotor activity based on broad optogenetic manipulations. However, because of the difficulties involved in accessing brainstem structures for in vivo cell type-specific recordings, V2a neuron function has never been directly observed during natural behaviors. Here, we imaged the activity of V2a neurons using micro-endoscopy in freely moving mice. We find that as many as half of the V2a neurons are excited at locomotion arrest and with low reliability. Other V2a neurons are inhibited at locomotor arrests and/or activated during other behaviors such as locomotion initiation or stationary grooming. Our results establish that V2a neurons not only drive stops as suggested by bulk optogenetics but also are stratified into subpopulations that likely contribute to diverse motor patterns.
Copyright © 2020 The Authors, some rights reserved; exclusive licensee American Association for the Advancement of Science. No claim to original U.S. Government Works. Distributed under a Creative Commons Attribution License 4.0 (CC BY).
Figures
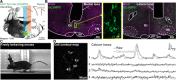
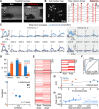
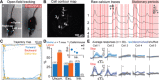
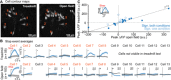
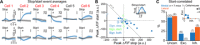
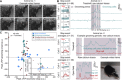
Similar articles
-
Control of Orienting Movements and Locomotion by Projection-Defined Subsets of Brainstem V2a Neurons.Curr Biol. 2020 Dec 7;30(23):4665-4681.e6. doi: 10.1016/j.cub.2020.09.014. Epub 2020 Oct 1. Curr Biol. 2020. PMID: 33007251
-
Descending Command Neurons in the Brainstem that Halt Locomotion.Cell. 2015 Nov 19;163(5):1191-1203. doi: 10.1016/j.cell.2015.10.074. Cell. 2015. PMID: 26590422 Free PMC article.
-
Hindbrain V2a neurons in the excitation of spinal locomotor circuits during zebrafish swimming.Curr Biol. 2013 May 20;23(10):843-9. doi: 10.1016/j.cub.2013.03.066. Epub 2013 Apr 25. Curr Biol. 2013. PMID: 23623549
-
Chx10+V2a interneurons in spinal motor regulation and spinal cord injury.Neural Regen Res. 2023 May;18(5):933-939. doi: 10.4103/1673-5374.355746. Neural Regen Res. 2023. PMID: 36254971 Free PMC article. Review.
-
Initiation of locomotion in lampreys.Brain Res Rev. 2008 Jan;57(1):172-82. doi: 10.1016/j.brainresrev.2007.07.016. Epub 2007 Aug 22. Brain Res Rev. 2008. PMID: 17916380 Review.
Cited by
-
The mesencephalic locomotor region recruits V2a reticulospinal neurons to drive forward locomotion in larval zebrafish.Nat Neurosci. 2023 Oct;26(10):1775-1790. doi: 10.1038/s41593-023-01418-0. Epub 2023 Sep 4. Nat Neurosci. 2023. PMID: 37667039 Free PMC article.
-
Hindbrain modules differentially transform activity of single collicular neurons to coordinate movements.Cell. 2023 Jul 6;186(14):3062-3078.e20. doi: 10.1016/j.cell.2023.05.031. Epub 2023 Jun 20. Cell. 2023. PMID: 37343561 Free PMC article.
-
Lumbar V3 interneurons provide direct excitatory synaptic input onto thoracic sympathetic preganglionic neurons, linking locomotor, and autonomic spinal systems.Front Neural Circuits. 2023 Aug 28;17:1235181. doi: 10.3389/fncir.2023.1235181. eCollection 2023. Front Neural Circuits. 2023. PMID: 37701071 Free PMC article.
-
Basal ganglia-spinal cord pathway that commands locomotor gait asymmetries in mice.Nat Neurosci. 2024 Apr;27(4):716-727. doi: 10.1038/s41593-024-01569-8. Epub 2024 Feb 12. Nat Neurosci. 2024. PMID: 38347200 Free PMC article.
-
Freely Behaving Mice Can Brake and Turn During Optogenetic Stimulation of the Mesencephalic Locomotor Region.Front Neural Circuits. 2021 Apr 9;15:639900. doi: 10.3389/fncir.2021.639900. eCollection 2021. Front Neural Circuits. 2021. PMID: 33897379 Free PMC article.
References
-
- Kiehn O., Development and functional organization of spinal locomotor circuits. Curr. Opin. Neurobiol. 21, 100–109 (2011). - PubMed
-
- Grillner S., El Manira A., Current principles of motor control, with special reference to vertebrate locomotion. Physiol. Rev. 100, 271–320 (2020). - PubMed
-
- Jordan L. M., Liu J., Hedlund P. B., Akay T., Pearson K. G., Descending command systems for the initiation of locomotion in mammals. Brain Res. Rev. 57, 183–191 (2008). - PubMed
Publication types
LinkOut - more resources
Full Text Sources