A Comparison of the Primary Sensory Neurons Used in Olfaction and Vision
- PMID: 33250719
- PMCID: PMC7676898
- DOI: 10.3389/fncel.2020.595523
A Comparison of the Primary Sensory Neurons Used in Olfaction and Vision
Abstract
Vision, hearing, smell, taste, and touch are the tools used to perceive and navigate the world. They enable us to obtain essential resources such as food and highly desired resources such as mates. Thanks to the investments in biomedical research the molecular unpinning's of human sensation are rivaled only by our knowledge of sensation in the laboratory mouse. Humans rely heavily on vision whereas mice use smell as their dominant sense. Both modalities have many features in common, starting with signal detection by highly specialized primary sensory neurons-rod and cone photoreceptors (PR) for vision, and olfactory sensory neurons (OSN) for the smell. In this chapter, we provide an overview of how these two types of primary sensory neurons operate while highlighting the similarities and distinctions.
Keywords: GPCR; olfaction; olfactory sensory neuron; photoreceptor; ribbon synapse; sensory receptors; vision; voltage-gated ion channel.
Copyright © 2020 Lankford, Laird, Inamdar and Baker.
Figures
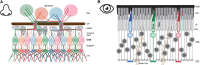
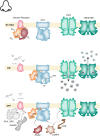
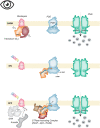
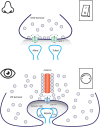
Similar articles
-
Sensory Transduction in Photoreceptors and Olfactory Sensory Neurons: Common Features and Distinct Characteristics.Front Cell Neurosci. 2021 Oct 8;15:761416. doi: 10.3389/fncel.2021.761416. eCollection 2021. Front Cell Neurosci. 2021. PMID: 34690705 Free PMC article. Review.
-
Engineering Aspects of Olfaction.In: Persaud KC, Marco S, Gutiérrez-Gálvez A, editors. Neuromorphic Olfaction. Boca Raton (FL): CRC Press/Taylor & Francis; 2013. Chapter 1. In: Persaud KC, Marco S, Gutiérrez-Gálvez A, editors. Neuromorphic Olfaction. Boca Raton (FL): CRC Press/Taylor & Francis; 2013. Chapter 1. PMID: 26042329 Free Books & Documents. Review.
-
The Synthetic Moth: A Neuromorphic Approach toward Artificial Olfaction in Robots.In: Persaud KC, Marco S, Gutiérrez-Gálvez A, editors. Neuromorphic Olfaction. Boca Raton (FL): CRC Press/Taylor & Francis; 2013. Chapter 4. In: Persaud KC, Marco S, Gutiérrez-Gálvez A, editors. Neuromorphic Olfaction. Boca Raton (FL): CRC Press/Taylor & Francis; 2013. Chapter 4. PMID: 26042327 Free Books & Documents. Review.
-
Mental Reactivation and Pleasantness Judgment of Experience Related to Vision, Hearing, Skin Sensations, Taste and Olfaction.PLoS One. 2016 Jul 11;11(7):e0159036. doi: 10.1371/journal.pone.0159036. eCollection 2016. PLoS One. 2016. PMID: 27400090 Free PMC article.
-
Anatomy of the olfactory system.Handb Clin Neurol. 2019;164:17-28. doi: 10.1016/B978-0-444-63855-7.00002-2. Handb Clin Neurol. 2019. PMID: 31604545 Review.
Cited by
-
Synaptic vesicle release during ribbon synapse formation of cone photoreceptors.Front Cell Neurosci. 2022 Nov 4;16:1022419. doi: 10.3389/fncel.2022.1022419. eCollection 2022. Front Cell Neurosci. 2022. PMID: 36406751 Free PMC article.
-
Effects of adenosine receptor overexpression and silencing in neurons and glial cells on lifespan, fitness, and sleep of Drosophila melanogaster.Exp Brain Res. 2023 Jul;241(7):1887-1904. doi: 10.1007/s00221-023-06649-y. Epub 2023 Jun 19. Exp Brain Res. 2023. PMID: 37335362 Free PMC article.
-
Mouse all-cone retina models of Cav1.4 synaptopathy.Front Mol Neurosci. 2023 Apr 27;16:1155955. doi: 10.3389/fnmol.2023.1155955. eCollection 2023. Front Mol Neurosci. 2023. PMID: 37181655 Free PMC article.
-
Sensory Transduction in Photoreceptors and Olfactory Sensory Neurons: Common Features and Distinct Characteristics.Front Cell Neurosci. 2021 Oct 8;15:761416. doi: 10.3389/fncel.2021.761416. eCollection 2021. Front Cell Neurosci. 2021. PMID: 34690705 Free PMC article. Review.
-
Nanotechnological approaches for efficient N2B delivery: from small-molecule drugs to biopharmaceuticals.Beilstein J Nanotechnol. 2024 Nov 12;15:1400-1414. doi: 10.3762/bjnano.15.113. eCollection 2024. Beilstein J Nanotechnol. 2024. PMID: 39559726 Free PMC article. Review.
References
Publication types
Grants and funding
LinkOut - more resources
Full Text Sources
Research Materials