Inactivation-mimicking block of the epithelial calcium channel TRPV6
- PMID: 33246965
- PMCID: PMC7695471
- DOI: 10.1126/sciadv.abe1508
Inactivation-mimicking block of the epithelial calcium channel TRPV6
Abstract
Epithelial calcium channel TRPV6 plays vital roles in calcium homeostasis, and its dysregulation is implicated in multifactorial diseases, including cancers. Here, we study the molecular mechanism of selective nanomolar-affinity TRPV6 inhibition by (4-phenylcyclohexyl)piperazine derivatives (PCHPDs). We use x-ray crystallography and cryo-electron microscopy to solve the inhibitor-bound structures of TRPV6 and identify two types of inhibitor binding sites in the transmembrane region: (i) modulatory sites between the S1-S4 and pore domains normally occupied by lipids and (ii) the main site in the ion channel pore. Our structural data combined with mutagenesis, functional and computational approaches suggest that PCHPDs plug the open pore of TRPV6 and convert the channel into a nonconducting state, mimicking the action of calmodulin, which causes inactivation of TRPV6 channels under physiological conditions. This mechanism of inhibition explains the high selectivity and potency of PCHPDs and opens up unexplored avenues for the design of future-generation biomimetic drugs.
Copyright © 2020 The Authors, some rights reserved; exclusive licensee American Association for the Advancement of Science. No claim to original U.S. Government Works. Distributed under a Creative Commons Attribution NonCommercial License 4.0 (CC BY-NC).
Figures
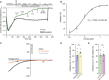
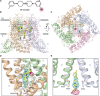
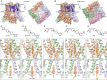
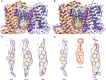
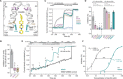
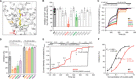
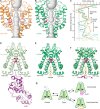
Similar articles
-
Mechanism of calmodulin inactivation of the calcium-selective TRP channel TRPV6.Sci Adv. 2018 Aug 15;4(8):eaau6088. doi: 10.1126/sciadv.aau6088. eCollection 2018 Aug. Sci Adv. 2018. PMID: 30116787 Free PMC article.
-
Structure and function of the calcium-selective TRP channel TRPV6.J Physiol. 2021 May;599(10):2673-2697. doi: 10.1113/JP279024. Epub 2020 Mar 13. J Physiol. 2021. PMID: 32073143 Free PMC article. Review.
-
Determining the Crystal Structure of TRPV6.In: Kozak JA, Putney JW Jr, editors. Calcium Entry Channels in Non-Excitable Cells. Boca Raton (FL): CRC Press/Taylor & Francis; 2018. Chapter 14. In: Kozak JA, Putney JW Jr, editors. Calcium Entry Channels in Non-Excitable Cells. Boca Raton (FL): CRC Press/Taylor & Francis; 2018. Chapter 14. PMID: 30299652 Free Books & Documents. Review.
-
Opening of the human epithelial calcium channel TRPV6.Nature. 2018 Jan 11;553(7687):233-237. doi: 10.1038/nature25182. Epub 2017 Dec 20. Nature. 2018. PMID: 29258289 Free PMC article.
-
Structural bases of TRP channel TRPV6 allosteric modulation by 2-APB.Nat Commun. 2018 Jun 25;9(1):2465. doi: 10.1038/s41467-018-04828-y. Nat Commun. 2018. PMID: 29941865 Free PMC article.
Cited by
-
Molecular pharmacology of the onco-TRP channel TRPV6.Channels (Austin). 2023 Dec;17(1):2266669. doi: 10.1080/19336950.2023.2266669. Epub 2023 Oct 15. Channels (Austin). 2023. PMID: 37838981 Free PMC article. Review.
-
Ligand-Binding Sites in Vanilloid-Subtype TRP Channels.Front Pharmacol. 2022 May 16;13:900623. doi: 10.3389/fphar.2022.900623. eCollection 2022. Front Pharmacol. 2022. PMID: 35652046 Free PMC article. Review.
-
Calcium Signalling in Breast Cancer Associated Bone Pain.Int J Mol Sci. 2022 Feb 8;23(3):1902. doi: 10.3390/ijms23031902. Int J Mol Sci. 2022. PMID: 35163823 Free PMC article. Review.
-
What structures did, and did not, reveal about the function of the epithelial Ca2+ channels TRPV5 and TRPV6.Cell Calcium. 2022 Sep;106:102620. doi: 10.1016/j.ceca.2022.102620. Epub 2022 Jul 3. Cell Calcium. 2022. PMID: 35834842 Free PMC article. Review.
-
Dynamic molecular portraits of ion-conducting pores characterize functional states of TRPV channels.Commun Chem. 2024 Jun 1;7(1):119. doi: 10.1038/s42004-024-01198-z. Commun Chem. 2024. PMID: 38824263 Free PMC article.
References
-
- Clapham D. E., TRP channels as cellular sensors. Nature 426, 517–524 (2003). - PubMed
-
- Nilius B., Szallasi A., Transient receptor potential channels as drug targets: From the science of basic research to the art of medicine. Pharmacol. Rev. 66, 676–814 (2014). - PubMed
-
- Bianco S. D., Peng J. B., Takanaga H., Suzuki Y., Crescenzi A., Kos C. H., Zhuang L., Freeman M. R., Gouveia C. H., Wu J., Luo H., Mauro T., Brown E. M., Hediger M. A., Marked disturbance of calcium homeostasis in mice with targeted disruption of the Trpv6 calcium channel gene. J. Bone Miner. Res. 22, 274–285 (2007). - PMC - PubMed
MeSH terms
Substances
Grants and funding
LinkOut - more resources
Full Text Sources