Oxycodone in the Opioid Epidemic: High 'Liking', 'Wanting', and Abuse Liability
- PMID: 33245509
- PMCID: PMC8155122
- DOI: 10.1007/s10571-020-01013-y
Oxycodone in the Opioid Epidemic: High 'Liking', 'Wanting', and Abuse Liability
Abstract
It is estimated that nearly a third of people who abuse drugs started with prescription opioid medicines. Approximately, 11.5 million Americans used prescription drugs recreationally in 2016, and in 2018, 46,802 Americans died as the result of an opioid overdose, including prescription opioids, heroin, and illicitly manufactured fentanyl (National Institutes on Drug Abuse (2020) Opioid Overdose Crisis. https://www.drugabuse.gov/drugs-abuse/opioids/opioid-overdose-crisis . Accessed 06 June 2020). Yet physicians will continue to prescribe oral opioids for moderate-to-severe pain in the absence of alternative therapeutics, underscoring the importance in understanding how drug choice can influence detrimental outcomes. One of the opioid prescription medications that led to this crisis is oxycodone, where misuse of this drug has been rampant. Being one of the most highly prescribed opioid medications for treating moderate-to-severe pain as reflected in the skyrocketed increase in retail sales of 866% between 1997 and 2007, oxycodone was initially suggested to be less addictive than morphine. The false-claimed non-addictive formulation of oxycodone, OxyContin, further contributed to the opioid crisis. Abuse was often carried out by crushing the pills for immediate burst release, typically by nasal insufflation, or by liquefying the pills for intravenous injection. Here, we review oxycodone pharmacology and abuse liability as well as present the hypothesis that oxycodone may exhibit a unique pharmacology that contributes to its high likability and abuse susceptibility. We will discuss various mechanisms that likely contribute to the high abuse rate of oxycodone including clinical drug likability, pharmacokinetics, pharmacodynamics, differences in its actions within mesolimbic reward circuity compared to other opioids, and the possibility of differential molecular and cellular receptor interactions that contribute to its selective effects. We will also discuss marketing strategies and drug difference that likely contributes to the oxycodone opioid use disorders and addiction.
Keywords: Allosteric site; Dopamine; Incentive salience; Likability; Oxycodone.
Conflict of interest statement
Figures
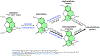
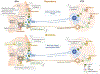
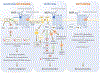
Similar articles
-
Abuse-Deterrent Formulations and the Prescription Opioid Abuse Epidemic in the United States: Lessons Learned From OxyContin.JAMA Psychiatry. 2015 May;72(5):424-30. doi: 10.1001/jamapsychiatry.2014.3043. JAMA Psychiatry. 2015. PMID: 25760692
-
Oxycodone's Unparalleled Addictive Potential: Is it Time for a Moratorium?Curr Pain Headache Rep. 2019 Feb 28;23(2):15. doi: 10.1007/s11916-019-0751-7. Curr Pain Headache Rep. 2019. PMID: 30820686
-
Online Conversation Monitoring to Understand the Opioid Epidemic: Epidemiological Surveillance Study.JMIR Public Health Surveill. 2020 Jun 29;6(2):e17073. doi: 10.2196/17073. JMIR Public Health Surveill. 2020. PMID: 32597786 Free PMC article.
-
Opioid formulations designed to resist/deter abuse.Drugs. 2010 Sep 10;70(13):1657-75. doi: 10.2165/11537940-000000000-00000. Drugs. 2010. PMID: 20731474 Review.
-
Likeability and abuse liability of commonly prescribed opioids.J Med Toxicol. 2012 Dec;8(4):335-40. doi: 10.1007/s13181-012-0263-x. J Med Toxicol. 2012. PMID: 22992943 Free PMC article. Review.
Cited by
-
Dissociable effects of oxycodone on behavior, calcium transient activity, and excitability of dorsolateral striatal neurons.Front Neural Circuits. 2022 Oct 26;16:983323. doi: 10.3389/fncir.2022.983323. eCollection 2022. Front Neural Circuits. 2022. PMID: 36389179 Free PMC article.
-
Effects of sex and estrous cycle on intravenous oxycodone self-administration and the reinstatement of oxycodone-seeking behavior in rats.Front Behav Neurosci. 2023 Jul 3;17:1143373. doi: 10.3389/fnbeh.2023.1143373. eCollection 2023. Front Behav Neurosci. 2023. PMID: 37465001 Free PMC article.
-
Opioid analgesic effects on subjective well-being in the operating theatre.Anaesthesia. 2023 Sep;78(9):1102-1111. doi: 10.1111/anae.16069. Epub 2023 Jun 28. Anaesthesia. 2023. PMID: 37381617 Free PMC article.
-
Oxycodone: A Current Perspective on Its Pharmacology, Abuse, and Pharmacotherapeutic Developments.Pharmacol Rev. 2023 Nov;75(6):1062-1118. doi: 10.1124/pharmrev.121.000506. Epub 2023 Jun 15. Pharmacol Rev. 2023. PMID: 37321860 Free PMC article. Review.
-
Sex differences in the rodent hippocampal opioid system following stress and oxycodone associated learning processes.Pharmacol Biochem Behav. 2022 Jan;212:173294. doi: 10.1016/j.pbb.2021.173294. Epub 2021 Nov 6. Pharmacol Biochem Behav. 2022. PMID: 34752798 Free PMC article. Review.
References
-
- Altarifi AA, David B, Muchhala KH, Blough BE, Akbarali H, Negus SS (2017) Effects of acute and repeated treatment with the biased mu opioid receptor agonist TRV130 (oliceridine) on measures of antinociception, gastrointestinal function, and abuse liability in rodents. J Psychopharmacol 31(6):730–739. 10.1177/0269881116689257 - DOI - PMC - PubMed