Theta-burst transcranial magnetic stimulation promotes stroke recovery by vascular protection and neovascularization
- PMID: 33204331
- PMCID: PMC7667689
- DOI: 10.7150/thno.51573
Theta-burst transcranial magnetic stimulation promotes stroke recovery by vascular protection and neovascularization
Abstract
Rationale: The integrity and function of the blood-brain barrier (BBB) is compromised after stroke. The current study was performed to examine potential beneficial effects and underlying mechanisms of repetitive transcranial magnetic stimulation (rTMS) on angiogenesis and vascular protection, function, and repair following stroke, which are largely unknown. Methods: Using a rat photothrombotic (PT) stroke model, continuous theta-burst rTMS was administered once daily to the infarcted hemisphere for 5 min, beginning 3 h after PT stroke. This treatment was applied for 6 days. BBB integrity, blood flow, vascular associated proteins, angiogenesis, integrity of neuronal morphology and structure, and behavioral outcome were measured and analyzed at 6 and/or 22 days after PT stroke. Results: We report that rTMS significantly mitigated BBB permeabilization and preserved important BBB components ZO-1, claudin-5, occludin, and caveolin-1 from PT-induced degradation. Damage to vascular structure, morphology, and perfusion was ameliorated by rTMS, resulting in improved local tissue oxygenation. This was accompanied with robust protection of critical vascular components and upregulation of regulatory factors. A complex cytokine response was induced by PT, particularly at the late phase. Application of rTMS modulated this response, ameliorating levels of cytokines related to peripheral immune cell infiltration. Further investigation revealed that rTMS promoted and sustained post-ischemic angiogenesis long-term and reduced apoptosis of newborn and existing vascular endothelial cells. Application of rTMS also inhibited PT-induced excessive astrocyte-vasculature interactions and stimulated an A1 to A2 shift in vessel-associated astrocytes. Mechanistic studies revealed that rTMS dramatically increased levels of PDGFRβ associated with A2 astrocytes and their adjacent vasculature. As well, A2 astrocytes displayed marked amplification of the angiogenesis-related factors VEGF and TGFβ. PT induced a rise in vessel-associated expression of HIF-1α that was starkly intensified by rTMS treatment. Finally, rTMS preserved neuronal morphology, synaptic structure integrity and behavioral outcome. Conclusions: These results indicate that rTMS can exert powerful protective and restorative effects on the peri-infarct microvasculature after PT stroke by, in part, promoting HIF-1α signaling and shifting vessel-associated astrocytic polarization to the A2 phenotype. This study provides further support for the potent protective effects of rTMS in the context of ischemic stroke, and these findings implicate vascular repair and protection as an important underlying phenomenon.
Keywords: HIF-1α; Transcranial Magnetic Stimulation (rTMS); angiogenesis; ischemic stroke; vascular protection; vascular repair.
© The author(s).
Conflict of interest statement
Competing Interests: The authors have declared that no competing interest exists.
Figures
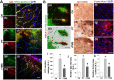
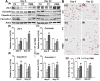
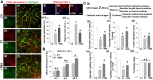
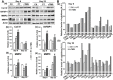
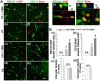
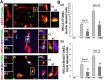
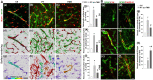
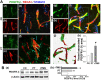
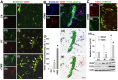
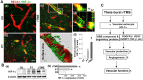
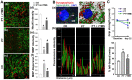
Similar articles
-
Continuous theta-burst stimulation enhances and sustains neurogenesis following ischemic stroke.Theranostics. 2022 Jul 18;12(13):5710-5726. doi: 10.7150/thno.71832. eCollection 2022. Theranostics. 2022. PMID: 35966576 Free PMC article.
-
Beneficial Effects of Theta-Burst Transcranial Magnetic Stimulation on Stroke Injury via Improving Neuronal Microenvironment and Mitochondrial Integrity.Transl Stroke Res. 2020 Jun;11(3):450-467. doi: 10.1007/s12975-019-00731-w. Epub 2019 Sep 12. Transl Stroke Res. 2020. PMID: 31515743
-
Repetitive Transcranial Magnetic Stimulation of the Brain After Ischemic Stroke: Mechanisms from Animal Models.Cell Mol Neurobiol. 2023 May;43(4):1487-1497. doi: 10.1007/s10571-022-01264-x. Epub 2022 Aug 2. Cell Mol Neurobiol. 2023. PMID: 35917043 Review.
-
High-frequency repetitive transcranial magnetic stimulation improves functional recovery by inhibiting neurotoxic polarization of astrocytes in ischemic rats.J Neuroinflammation. 2020 May 6;17(1):150. doi: 10.1186/s12974-020-01747-y. J Neuroinflammation. 2020. PMID: 32375835 Free PMC article.
-
Noninvasive Brain Stimulation to Enhance Functional Recovery After Stroke: Studies in Animal Models.Neurorehabil Neural Repair. 2018 Nov;32(11):927-940. doi: 10.1177/1545968318804425. Epub 2018 Oct 24. Neurorehabil Neural Repair. 2018. PMID: 30352528 Free PMC article. Review.
Cited by
-
Activation of testosterone-androgen receptor mediates cerebrovascular protection by photobiomodulation treatment in photothrombosis-induced stroke rats.CNS Neurosci Ther. 2024 Feb;30(2):e14574. doi: 10.1111/cns.14574. CNS Neurosci Ther. 2024. PMID: 38421088 Free PMC article.
-
Low intensity repetitive transcranial magnetic stimulation enhances remyelination by newborn and surviving oligodendrocytes in the cuprizone model of toxic demyelination.Cell Mol Life Sci. 2024 Aug 12;81(1):346. doi: 10.1007/s00018-024-05391-0. Cell Mol Life Sci. 2024. PMID: 39134808 Free PMC article.
-
Systematic review and meta-analysis of repetitive transcranial magnetic stimulation (rTMS) for activities of daily living in Alzheimer's disease.Neurol Sci. 2025 Jan;46(1):63-77. doi: 10.1007/s10072-024-07709-z. Epub 2024 Jul 23. Neurol Sci. 2025. PMID: 39044102 Review.
-
The Implications of Microglial Regulation in Neuroplasticity-Dependent Stroke Recovery.Biomolecules. 2023 Mar 21;13(3):571. doi: 10.3390/biom13030571. Biomolecules. 2023. PMID: 36979506 Free PMC article. Review.
-
Continuous theta-burst stimulation enhances and sustains neurogenesis following ischemic stroke.Theranostics. 2022 Jul 18;12(13):5710-5726. doi: 10.7150/thno.71832. eCollection 2022. Theranostics. 2022. PMID: 35966576 Free PMC article.
References
-
- Benjamin EJ, Muntner P, Alonso A, Bittencourt MS, Callaway CW, Carson AP. et al. Heart Disease and Stroke Statistics-2019 Update: A Report From the American Heart Association. Circulation. 2019;139:e56–e528. - PubMed
-
- Derex L, Cho TH. Mechanical thrombectomy in acute ischemic stroke. Rev Neurol (Paris) 2017;173:106–13. - PubMed
-
- Hofmeijer J, van Putten MJ. Ischemic cerebral damage: an appraisal of synaptic failure. Stroke. 2012;43:607–15. - PubMed
Publication types
MeSH terms
LinkOut - more resources
Full Text Sources
Medical
Research Materials