tRNA Fragments Populations Analysis in Mutants Affecting tRNAs Processing and tRNA Methylation
- PMID: 33193603
- PMCID: PMC7586317
- DOI: 10.3389/fgene.2020.518949
tRNA Fragments Populations Analysis in Mutants Affecting tRNAs Processing and tRNA Methylation
Abstract
tRNA fragments (tRFs) are a class of small non-coding RNAs (sncRNAs) derived from tRNAs. tRFs are highly abundant in many cell types including stem cells and cancer cells, and are found in all domains of life. Beyond translation control, tRFs have several functions ranging from transposon silencing to cell proliferation control. However, the analysis of tRFs presents specific challenges and their biogenesis is not well understood. They are very heterogeneous and highly modified by numerous post-transcriptional modifications. Here we describe a bioinformatic pipeline (tRFs-Galaxy) to study tRFs populations and shed light onto tRNA fragments biogenesis in Drosophila melanogaster. Indeed, we used small RNAs Illumina sequencing datasets extracted from wild type and mutant ovaries affecting two different highly conserved steps of tRNA biogenesis: 5'pre-tRNA processing (RNase-P subunit Rpp30) and tRNA 2'-O-methylation (dTrm7_34 and dTrm7_32). Using our pipeline, we show how defects in tRNA biogenesis affect nuclear and mitochondrial tRFs populations and other small non-coding RNAs biogenesis, such as small nucleolar RNAs (snoRNAs). This tRF analysis workflow will advance the current understanding of tRFs biogenesis, which is crucial to better comprehend tRFs roles and their implication in human pathology.
Keywords: Drosophila; Nm methylation; RNase P; oogenesis; tRFs; tRNA.
Copyright © 2020 Molla-Herman, Angelova, Ginestet, Carré, Antoniewski and Huynh.
Figures
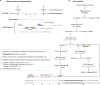
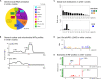
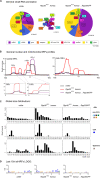
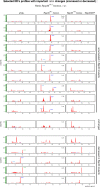
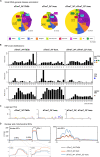
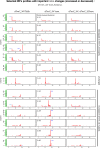
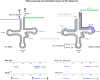
Similar articles
-
Extracellular vesicles-associated tRNA-derived fragments (tRFs): biogenesis, biological functions, and their role as potential biomarkers in human diseases.J Mol Med (Berl). 2022 May;100(5):679-695. doi: 10.1007/s00109-022-02189-0. Epub 2022 Mar 24. J Mol Med (Berl). 2022. PMID: 35322869 Free PMC article. Review.
-
Genome-wide identification and characterization of tRNA-derived RNA fragments in land plants.Plant Mol Biol. 2017 Jan;93(1-2):35-48. doi: 10.1007/s11103-016-0545-9. Epub 2016 Sep 28. Plant Mol Biol. 2017. PMID: 27681945
-
The Expression Pattern of tRNA-Derived Small RNAs in Adult Drosophila and the Function of tRF-Trp-CCA-014-H3C4 Network Analysis.Int J Mol Sci. 2023 Mar 24;24(7):6169. doi: 10.3390/ijms24076169. Int J Mol Sci. 2023. PMID: 37047149 Free PMC article.
-
A novel class of small RNAs: tRNA-derived RNA fragments (tRFs).Genes Dev. 2009 Nov 15;23(22):2639-49. doi: 10.1101/gad.1837609. Genes Dev. 2009. PMID: 19933153 Free PMC article.
-
Emerging Classes of Small Non-Coding RNAs With Potential Implications in Diabetes and Associated Metabolic Disorders.Front Endocrinol (Lausanne). 2021 May 10;12:670719. doi: 10.3389/fendo.2021.670719. eCollection 2021. Front Endocrinol (Lausanne). 2021. PMID: 34040585 Free PMC article. Review.
Cited by
-
Inosine in Biology and Disease.Genes (Basel). 2021 Apr 19;12(4):600. doi: 10.3390/genes12040600. Genes (Basel). 2021. PMID: 33921764 Free PMC article. Review.
-
Cardiac RNase Z edited via CRISPR-Cas9 drives heart hypertrophy in Drosophila.PLoS One. 2023 May 25;18(5):e0286214. doi: 10.1371/journal.pone.0286214. eCollection 2023. PLoS One. 2023. PMID: 37228086 Free PMC article.
-
"Transfer" of power: The intersection of DNA virus infection and tRNA biology.Semin Cell Dev Biol. 2023 Sep 15;146:31-39. doi: 10.1016/j.semcdb.2023.01.011. Epub 2023 Jan 20. Semin Cell Dev Biol. 2023. PMID: 36682929 Free PMC article. Review.
-
Gly-tRF enhances LCSC-like properties and promotes HCC cells migration by targeting NDFIP2.Cancer Cell Int. 2021 Sep 18;21(1):502. doi: 10.1186/s12935-021-02102-8. Cancer Cell Int. 2021. PMID: 34537070 Free PMC article.
-
Quantification of substoichiometric modification reveals global tsRNA hypomodification, preferences for angiogenin-mediated tRNA cleavage, and idiosyncratic epitranscriptomes of human neuronal cell-lines.Comput Struct Biotechnol J. 2022 Dec 19;21:401-417. doi: 10.1016/j.csbj.2022.12.020. eCollection 2023. Comput Struct Biotechnol J. 2022. PMID: 36618980 Free PMC article.
References
LinkOut - more resources
Full Text Sources
Molecular Biology Databases