Priming Astrocytes With HIV-Induced Reactive Oxygen Species Enhances Their Trypanosoma cruzi Infection
- PMID: 33193149
- PMCID: PMC7604310
- DOI: 10.3389/fmicb.2020.563320
Priming Astrocytes With HIV-Induced Reactive Oxygen Species Enhances Their Trypanosoma cruzi Infection
Abstract
Introduction: Trypanosoma cruzi is an intracellular protozoa and etiological agent that causes Chagas disease. Its presence among the immunocompromised HIV-infected individuals is relevant worldwide because of its impact on the central nervous system (CNS) causing severe meningoencephalitis. The HIV infection of astrocytes - the most abundant cells in the brain, where the parasite can also be hosted - being able to modify reactive oxygen species (ROS) could influence the parasite growth. In such interaction, extracellular vesicles (EVs) shed from trypomastigotes may alter the surrounding environment including its pro-oxidant status. Methods: We evaluated the interplay between both pathogens in human astrocytes and its consequences on the host cell pro-oxidant condition self-propitiated by the parasite - using its EVs - or by HIV infection. For this goal, we challenged cultured human primary astrocytes with both pathogens and the efficiency of infection and multiplication were measured by microscopy and flow cytometry and parasite DNA quantification. Mitochondrial and cellular ROS levels were measured by flow cytometry in the presence or not of scavengers with a concomitant evaluation of the cellular apoptosis level. Results: We observed that increased mitochondrial and cellular ROS production boosted significantly T. cruzi infection and multiplication in astrocytes. Such oxidative condition was promoted by free trypomastigotes-derived EVs as well as by HIV infection. Conclusions: The pathogenesis of the HIV-T. cruzi coinfection in astrocytes leads to an oxidative misbalance as a key mechanism, which exacerbates ROS generation and promotes positive feedback to parasite growth in the CNS.
Keywords: Trypanosoma cruzi; astrocytes; extracellular vesicles; human immunodeficiency virus; reactive oxygen species.
Copyright © 2020 Urquiza, Cevallos, Elizalde, Delpino and Quarleri.
Figures
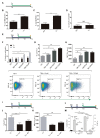
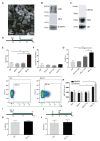
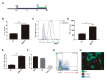
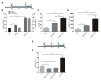
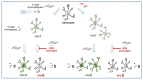
Similar articles
-
Astrocyte Apoptosis and HIV Replication Are Modulated in Host Cells Coinfected with Trypanosoma cruzi.Front Cell Infect Microbiol. 2017 Aug 2;7:345. doi: 10.3389/fcimb.2017.00345. eCollection 2017. Front Cell Infect Microbiol. 2017. PMID: 28824880 Free PMC article.
-
Priming astrocytes with TNF enhances their susceptibility to Trypanosoma cruzi infection and creates a self-sustaining inflammatory milieu.J Neuroinflammation. 2017 Sep 6;14(1):182. doi: 10.1186/s12974-017-0952-0. J Neuroinflammation. 2017. PMID: 28877735 Free PMC article.
-
Extracellular vesicles of Trypanosoma cruzi tissue-culture cell-derived trypomastigotes: Induction of physiological changes in non-parasitized culture cells.PLoS Negl Trop Dis. 2019 Feb 21;13(2):e0007163. doi: 10.1371/journal.pntd.0007163. eCollection 2019 Feb. PLoS Negl Trop Dis. 2019. PMID: 30789912 Free PMC article.
-
Extracellular Vesicles: Potential Role in Remote Signaling and Inflammation in Trypanosoma cruzi-Triggered Disease.Front Cell Dev Biol. 2021 Dec 20;9:798054. doi: 10.3389/fcell.2021.798054. eCollection 2021. Front Cell Dev Biol. 2021. PMID: 34988085 Free PMC article. Review.
-
How Trypanosoma cruzi deals with oxidative stress: Antioxidant defence and DNA repair pathways.Mutat Res Rev Mutat Res. 2016 Jan-Mar;767:8-22. doi: 10.1016/j.mrrev.2015.12.003. Epub 2015 Dec 29. Mutat Res Rev Mutat Res. 2016. PMID: 27036062 Review.
Cited by
-
Chagas disease in immunocompromised patients.Clin Microbiol Rev. 2024 Jun 13;37(2):e0009923. doi: 10.1128/cmr.00099-23. Epub 2024 Mar 28. Clin Microbiol Rev. 2024. PMID: 38546225 Review.
-
Chagas Disease in People with HIV: A Narrative Review.Trop Med Infect Dis. 2021 Nov 9;6(4):198. doi: 10.3390/tropicalmed6040198. Trop Med Infect Dis. 2021. PMID: 34842854 Free PMC article. Review.
-
Exploring Peripheral Blood-Derived Extracellular Vesicles as Biomarkers: Implications for Chronic Chagas Disease with Viral Infection or Transplantation.Microorganisms. 2024 Jan 5;12(1):116. doi: 10.3390/microorganisms12010116. Microorganisms. 2024. PMID: 38257943 Free PMC article.
References
-
- Arantes J. M., Pedrosa M. L., Martins H. R., Veloso V. M., de Lana M., Bahia M. T., et al. . (2007). Trypanosoma cruzi: treatment with the iron chelator desferrioxamine reduces parasitemia and mortality in experimentally infected mice. Exp. Parasitol. 117, 43–50. 10.1016/j.exppara.2007.03.006, PMID: - DOI - PubMed
LinkOut - more resources
Full Text Sources