An Overview on SARS-CoV-2 (COVID-19) and Other Human Coronaviruses and Their Detection Capability via Amplification Assay, Chemical Sensing, Biosensing, Immunosensing, and Clinical Assays
- PMID: 33163530
- PMCID: PMC7604542
- DOI: 10.1007/s40820-020-00533-y
An Overview on SARS-CoV-2 (COVID-19) and Other Human Coronaviruses and Their Detection Capability via Amplification Assay, Chemical Sensing, Biosensing, Immunosensing, and Clinical Assays
Abstract
A novel coronavirus of zoonotic origin (SARS-CoV-2) has recently been recognized in patients with acute respiratory disease. COVID-19 causative agent is structurally and genetically similar to SARS and bat SARS-like coronaviruses. The drastic increase in the number of coronavirus and its genome sequence have given us an unprecedented opportunity to perform bioinformatics and genomics analysis on this class of viruses. Clinical tests like PCR and ELISA for rapid detection of this virus are urgently needed for early identification of infected patients. However, these techniques are expensive and not readily available for point-of-care (POC) applications. Currently, lack of any rapid, available, and reliable POC detection method gives rise to the progression of COVID-19 as a horrible global problem. To solve the negative features of clinical investigation, we provide a brief introduction of the general features of coronaviruses and describe various amplification assays, sensing, biosensing, immunosensing, and aptasensing for the determination of various groups of coronaviruses applied as a template for the detection of SARS-CoV-2. All sensing and biosensing techniques developed for the determination of various classes of coronaviruses are useful to recognize the newly immerged coronavirus, i.e., SARS-CoV-2. Also, the introduction of sensing and biosensing methods sheds light on the way of designing a proper screening system to detect the virus at the early stage of infection to tranquilize the speed and vastity of spreading. Among other approaches investigated among molecular approaches and PCR or recognition of viral diseases, LAMP-based methods and LFAs are of great importance for their numerous benefits, which can be helpful to design a universal platform for detection of future emerging pathogenic viruses.
Keywords: Amplification assay; Apta assay; ELISA; Sensing assay; qRT-PCR.
© The Author(s) 2020.
Figures
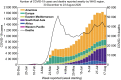
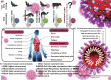
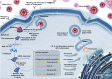
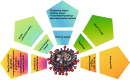
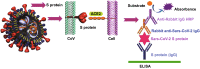
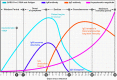
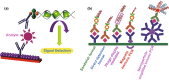
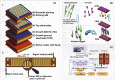
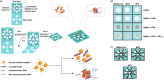
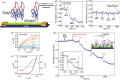
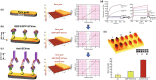
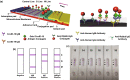
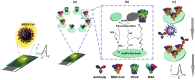
Similar articles
-
Development of a reverse transcription-loop-mediated isothermal amplification as a rapid early-detection method for novel SARS-CoV-2.Emerg Microbes Infect. 2020 Dec;9(1):998-1007. doi: 10.1080/22221751.2020.1756698. Emerg Microbes Infect. 2020. PMID: 32306853 Free PMC article.
-
Colorimetric reverse transcription loop-mediated isothermal amplification (RT-LAMP) as a visual diagnostic platform for the detection of the emerging coronavirus SARS-CoV-2.Analyst. 2021 Jan 21;146(2):471-477. doi: 10.1039/d0an01775b. Epub 2020 Nov 9. Analyst. 2021. PMID: 33165486
-
Loop mediated isothermal amplification (LAMP) assays as a rapid diagnostic for COVID-19.Med Hypotheses. 2020 Aug;141:109786. doi: 10.1016/j.mehy.2020.109786. Epub 2020 Apr 25. Med Hypotheses. 2020. PMID: 32361529 Free PMC article.
-
Current status of antivirals and druggable targets of SARS CoV-2 and other human pathogenic coronaviruses.Drug Resist Updat. 2020 Dec;53:100721. doi: 10.1016/j.drup.2020.100721. Epub 2020 Aug 26. Drug Resist Updat. 2020. PMID: 33132205 Free PMC article. Review.
-
Electrochemical SARS-CoV-2 Sensing at Point-of-Care and Artificial Intelligence for Intelligent COVID-19 Management.ACS Appl Bio Mater. 2020 Nov 16;3(11):7306-7325. doi: 10.1021/acsabm.0c01004. Epub 2020 Oct 27. ACS Appl Bio Mater. 2020. PMID: 35019473 Review.
Cited by
-
Gold Nanoconjugates for miRNA Modulation in Cancer Therapy: From miRNA Silencing to miRNA Mimics.ACS Mater Au. 2022 Nov 9;2(6):626-640. doi: 10.1021/acsmaterialsau.2c00042. Epub 2022 Aug 13. ACS Mater Au. 2022. PMID: 36397876 Free PMC article. Review.
-
Untethered Micro/Nanorobots for Remote Sensing: Toward Intelligent Platform.Nanomicro Lett. 2023 Nov 30;16(1):40. doi: 10.1007/s40820-023-01261-9. Nanomicro Lett. 2023. PMID: 38032461 Free PMC article. Review.
-
Electrochemical diagnostics of infectious viral diseases: Trends and challenges.Biosens Bioelectron. 2021 May 15;180:113112. doi: 10.1016/j.bios.2021.113112. Epub 2021 Mar 2. Biosens Bioelectron. 2021. PMID: 33706158 Free PMC article. Review.
-
Computational Method-Based Optimization of Carbon Nanotube Thin-Film Immunosensor for Rapid Detection of SARS-CoV-2 Virus.Small Sci. 2022 Feb;2(2):2100111. doi: 10.1002/smsc.202100111. Epub 2021 Nov 16. Small Sci. 2022. PMID: 34901932 Free PMC article.
-
Triple-target stimuli-responsive anti-COVID-19 face mask with physiological virus-inactivating agents.Biomater Sci. 2021 Sep 14;9(18):6052-6063. doi: 10.1039/d1bm00502b. Biomater Sci. 2021. PMID: 34190748 Free PMC article.
References
Publication types
LinkOut - more resources
Full Text Sources
Miscellaneous