Man-Specific, GalNAc/T/Tn-Specific and Neu5Ac-Specific Seaweed Lectins as Glycan Probes for the SARS-CoV-2 (COVID-19) Coronavirus
- PMID: 33138151
- PMCID: PMC7693892
- DOI: 10.3390/md18110543
Man-Specific, GalNAc/T/Tn-Specific and Neu5Ac-Specific Seaweed Lectins as Glycan Probes for the SARS-CoV-2 (COVID-19) Coronavirus
Abstract
Seaweed lectins, especially high-mannose-specific lectins from red algae, have been identified as potential antiviral agents that are capable of blocking the replication of various enveloped viruses like influenza virus, herpes virus, and HIV-1 in vitro. Their antiviral activity depends on the recognition of glycoprotein receptors on the surface of sensitive host cells-in particular, hemagglutinin for influenza virus or gp120 for HIV-1, which in turn triggers fusion events, allowing the entry of the viral genome into the cells and its subsequent replication. The diversity of glycans present on the S-glycoproteins forming the spikes covering the SARS-CoV-2 envelope, essentially complex type N-glycans and high-mannose type N-glycans, suggests that high-mannose-specific seaweed lectins are particularly well adapted as glycan probes for coronaviruses. This review presents a detailed study of the carbohydrate-binding specificity of high-mannose-specific seaweed lectins, demonstrating their potential to be used as specific glycan probes for coronaviruses, as well as the biomedical interest for both the detection and immobilization of SARS-CoV-2 to avoid shedding of the virus into the environment. The use of these seaweed lectins as replication blockers for SARS-CoV-2 is also discussed.
Keywords: COVID-19; N-acetylgalactosamine-specific lectins; N-glycosylation; O-glycosylation; SARS-CoV-2; T/Tn-specific lectins; glycan probes; griffithsin; high-mannose glycans; mannose-specific lectins; red algae; seaweed lectins.
Conflict of interest statement
The authors declare no conflict of interest.
Figures
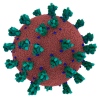
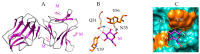
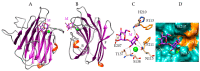
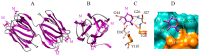
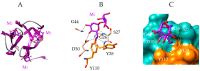
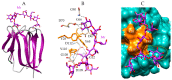
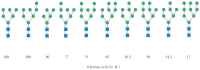
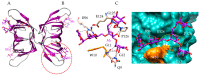
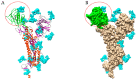
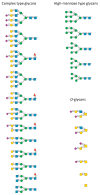
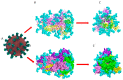
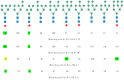
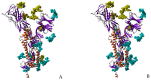
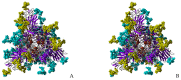
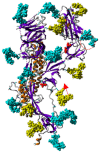
Similar articles
-
Legume Lectins with Different Specificities as Potential Glycan Probes for Pathogenic Enveloped Viruses.Cells. 2022 Jan 20;11(3):339. doi: 10.3390/cells11030339. Cells. 2022. PMID: 35159151 Free PMC article. Review.
-
Man-Specific Lectins from Plants, Fungi, Algae and Cyanobacteria, as Potential Blockers for SARS-CoV, MERS-CoV and SARS-CoV-2 (COVID-19) Coronaviruses: Biomedical Perspectives.Cells. 2021 Jun 28;10(7):1619. doi: 10.3390/cells10071619. Cells. 2021. PMID: 34203435 Free PMC article. Review.
-
SARS-CoV-2 Evolutionary Adaptation toward Host Entry and Recognition of Receptor O-Acetyl Sialylation in Virus-Host Interaction.Int J Mol Sci. 2020 Jun 26;21(12):4549. doi: 10.3390/ijms21124549. Int J Mol Sci. 2020. PMID: 32604730 Free PMC article. Review.
-
Plant lectins as versatile tools to fight coronavirus outbreaks.Glycoconj J. 2023 Feb;40(1):109-118. doi: 10.1007/s10719-022-10094-4. Epub 2022 Nov 24. Glycoconj J. 2023. PMID: 36418811 Free PMC article. Review.
-
Mannose-specific plant and microbial lectins as antiviral agents: A review.Glycoconj J. 2024 Feb;41(1):1-33. doi: 10.1007/s10719-023-10142-7. Epub 2024 Jan 20. Glycoconj J. 2024. PMID: 38244136 Review.
Cited by
-
PepTraq: a toolbox for in silico data mining and fast sequence filtering.Amino Acids. 2023 May;55(5):709-712. doi: 10.1007/s00726-023-03251-y. Epub 2023 Mar 8. Amino Acids. 2023. PMID: 36884076
-
Seaweed-Derived Proteins and Peptides: Promising Marine Bioactives.Antioxidants (Basel). 2022 Jan 17;11(1):176. doi: 10.3390/antiox11010176. Antioxidants (Basel). 2022. PMID: 35052680 Free PMC article. Review.
-
Fighting SARS-CoV-2 with green seaweed Ulva sp. extract: extraction protocol predetermines crude ulvan extract anti-SARS-CoV-2 inhibition properties in in vitro Vero-E6 cells assay.PeerJ. 2021 Nov 15;9:e12398. doi: 10.7717/peerj.12398. eCollection 2021. PeerJ. 2021. PMID: 34820178 Free PMC article.
-
New Hopes for Drugs against COVID-19 Come from the Sea.Mar Drugs. 2021 Feb 11;19(2):104. doi: 10.3390/md19020104. Mar Drugs. 2021. PMID: 33670191 Free PMC article.
-
Plant lectins as prospective antiviral biomolecules in the search for COVID-19 eradication strategies.Biomed Pharmacother. 2022 Feb;146:112507. doi: 10.1016/j.biopha.2021.112507. Epub 2021 Dec 7. Biomed Pharmacother. 2022. PMID: 34891122 Free PMC article. Review.
References
-
- Boyd W.C., Almodóvar L.R., Boyd L.G. Agglutinins in Marine Algae for Human Erythrocytes. Transfusion. 1966;6:82–83. doi: 10.1111/j.1537-2995.1966.tb04699.x. - DOI
-
- Blunden G., Rogers D.J., Farnham W.F. Survey of British Seaweeds for Hæmagglutinins. Lloydia. 1975;36:162–168. - PubMed
-
- Hori K., Ikegami S., Miyazawa K., Ito K. Mitogenic and Antineoplastic Isoagglutinins from the Red Alga Solieria robusta. Phytochemistry. 1988;27:2063–2067. doi: 10.1016/0031-9422(88)80097-9. - DOI
-
- Sugahara T., Ohama Y., Fukuda A., Hayashi M., Kawakubo A., Kato K. The Cytotoxic Effect of Eucheuma serrata Agglutinin (ESA) on Cancer Cells and Its Application to Molecular Probe for Drug Delivery System Using Lipid Vesicles. Cytotechnology. 2001;36:93–99. doi: 10.1023/A:1014057407251. - DOI - PMC - PubMed
Publication types
MeSH terms
Substances
LinkOut - more resources
Full Text Sources
Miscellaneous