Using Gene Editing Approaches to Fine-Tune the Immune System
- PMID: 33117361
- PMCID: PMC7553077
- DOI: 10.3389/fimmu.2020.570672
Using Gene Editing Approaches to Fine-Tune the Immune System
Abstract
Genome editing technologies not only provide unprecedented opportunities to study basic cellular system functionality but also improve the outcomes of several clinical applications. In this review, we analyze various gene editing techniques used to fine-tune immune systems from a basic research and clinical perspective. We discuss recent advances in the development of programmable nucleases, such as zinc-finger nucleases (ZFNs), transcription activator-like effector nucleases (TALENs), and clustered regularly interspaced short palindromic repeat (CRISPR)-Cas-associated nucleases. We also discuss the use of programmable nucleases and their derivative reagents such as base editing tools to engineer immune cells via gene disruption, insertion, and rewriting of T cells and other immune components, such natural killers (NKs) and hematopoietic stem and progenitor cells (HSPCs). In addition, with regard to chimeric antigen receptors (CARs), we describe how different gene editing tools enable healthy donor cells to be used in CAR T therapy instead of autologous cells without risking graft-versus-host disease or rejection, leading to reduced adoptive cell therapy costs and instant treatment availability for patients. We pay particular attention to the delivery of therapeutic transgenes, such as CARs, to endogenous loci which prevents collateral damage and increases therapeutic effectiveness. Finally, we review creative innovations, including immune system repurposing, that facilitate safe and efficient genome surgery within the framework of clinical cancer immunotherapies.
Keywords: CARs; base editors; gene editing; graft-vs-host disease; immunotherapy.
Copyright © 2020 Pavlovic, Tristán-Manzano, Maldonado-Pérez, Cortijo-Gutierrez, Sánchez-Hernández, Justicia-Lirio, Carmona, Herrera, Martin and Benabdellah.
Figures
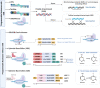
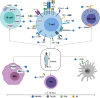
Similar articles
-
Gene editing and CRISPR in the clinic: current and future perspectives.Biosci Rep. 2020 Apr 30;40(4):BSR20200127. doi: 10.1042/BSR20200127. Biosci Rep. 2020. PMID: 32207531 Free PMC article. Review.
-
Retroviral Vectors for Cancer Gene Therapy.Recent Results Cancer Res. 2016;209:17-35. doi: 10.1007/978-3-319-42934-2_2. Recent Results Cancer Res. 2016. PMID: 28101685 Review.
-
INDEL detection, the 'Achilles heel' of precise genome editing: a survey of methods for accurate profiling of gene editing induced indels.Nucleic Acids Res. 2020 Dec 2;48(21):11958-11981. doi: 10.1093/nar/gkaa975. Nucleic Acids Res. 2020. PMID: 33170255 Free PMC article. Review.
-
Genome editing: the road of CRISPR/Cas9 from bench to clinic.Exp Mol Med. 2016 Oct 14;48(10):e265. doi: 10.1038/emm.2016.111. Exp Mol Med. 2016. PMID: 27741224 Free PMC article. Review.
-
Therapeutic potential of CRISPR/CAS9 genome modification in T cell-based immunotherapy of cancer.Cytotherapy. 2024 May;26(5):436-443. doi: 10.1016/j.jcyt.2024.02.014. Epub 2024 Feb 23. Cytotherapy. 2024. PMID: 38466263 Review.
Cited by
-
Dietary factors and their influence on immunotherapy strategies in oncology: a comprehensive review.Cell Death Dis. 2024 Apr 9;15(4):254. doi: 10.1038/s41419-024-06641-6. Cell Death Dis. 2024. PMID: 38594256 Free PMC article. Review.
-
Application of Genomic Medicine in Africa: 14th Conference of the African Society of Human Genetics and the 2nd International Congress of the Moroccan Society of Genomics and Human Genetics, Rabat, Morocco 2022.Am J Trop Med Hyg. 2024 May 2;110(6):1279-1284. doi: 10.4269/ajtmh.23-0808. Print 2024 Jun 5. Am J Trop Med Hyg. 2024. PMID: 38697089
-
Homology-independent targeted insertion-mediated derivation of M1-biased macrophages harbouring Megf10 and CD3ζ from human pluripotent stem cells.EBioMedicine. 2024 Nov;109:105390. doi: 10.1016/j.ebiom.2024.105390. Epub 2024 Oct 9. EBioMedicine. 2024. PMID: 39383607 Free PMC article.
-
Efficient Editing of the CXCR4 Locus Using Cas9 Ribonucleoprotein Complexes Stabilized with Polyglutamic Acid.Dokl Biol Sci. 2023 Dec;513(Suppl 1):S28-S32. doi: 10.1134/S0012496623700862. Epub 2024 Jan 8. Dokl Biol Sci. 2023. PMID: 38190037
-
From Descriptive to Functional Genomics of Leukemias Focusing on Genome Engineering Techniques.Int J Mol Sci. 2021 Sep 17;22(18):10065. doi: 10.3390/ijms221810065. Int J Mol Sci. 2021. PMID: 34576226 Free PMC article. Review.
References
Publication types
MeSH terms
Substances
LinkOut - more resources
Full Text Sources