Understanding Severe Acute Respiratory Syndrome Coronavirus 2 Replication to Design Efficient Drug Combination Therapies
- PMID: 33099545
- PMCID: PMC7649723
- DOI: 10.1159/000512141
Understanding Severe Acute Respiratory Syndrome Coronavirus 2 Replication to Design Efficient Drug Combination Therapies
Abstract
Background: The emergence of severe acute respiratory syndrome coronavirus 2 (SARS-CoV-2) and its disease CO-VID-19 has strongly encouraged the search for antiviral compounds. Most of the evaluated drugs against SARS-CoV-2 derive from drug repurposing of Food and Drug Administration-approved molecules. These drugs have as target three major processes: (1) early stages of virus-cell interaction, (2) viral proteases, and (3) the viral RNA-dependent RNA polymerase.
Summary: This review focused on the basic principles of virology and pharmacology to understand the importance of early stages of virus-cell interaction as therapeutic targets and other main processes vital for SARS-CoV-2 replication. Furthermore, we focused on describing the main targets associated with SARS-CoV-2 antiviral therapy and the rationale of drug combinations for efficiently suppressing viral replication. Key Messages: We hypothesized that blocking of both entry mechanisms could allow a more effective antiviral effect compared to the partial results obtained with chloroquine or its derivatives alone. This approach, already used to achieve an antiviral effect higher than that offered by every single drug administered separately, has been successfully applied in several viral infections such as HIV and HCV. This review will contribute to expanding the perception of the possible therapeutic targets in SARS-CoV-2 infection and highlight the benefits of using combination therapies.
Keywords: Combination; Coronavirus; Entry; Protease; SARS-CoV-2; Treatment; Virus-host interactions.
© 2020 S. Karger AG, Basel.
Conflict of interest statement
The authors have no conflicts of interest to declare.
Figures
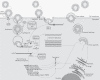
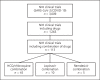
Similar articles
-
Cellular host factors for SARS-CoV-2 infection.Nat Microbiol. 2021 Oct;6(10):1219-1232. doi: 10.1038/s41564-021-00958-0. Epub 2021 Sep 1. Nat Microbiol. 2021. PMID: 34471255 Review.
-
Precision therapeutic targets for COVID-19.Virol J. 2021 Mar 29;18(1):66. doi: 10.1186/s12985-021-01526-y. Virol J. 2021. PMID: 33781287 Free PMC article. Review.
-
Mapping the SARS-CoV-2-Host Protein-Protein Interactome by Affinity Purification Mass Spectrometry and Proximity-Dependent Biotin Labeling: A Rational and Straightforward Route to Discover Host-Directed Anti-SARS-CoV-2 Therapeutics.Int J Mol Sci. 2021 Jan 7;22(2):532. doi: 10.3390/ijms22020532. Int J Mol Sci. 2021. PMID: 33430309 Free PMC article. Review.
-
TMPRSS2 and RNA-Dependent RNA Polymerase Are Effective Targets of Therapeutic Intervention for Treatment of COVID-19 Caused by SARS-CoV-2 Variants (B.1.1.7 and B.1.351).Microbiol Spectr. 2021 Sep 3;9(1):e0047221. doi: 10.1128/Spectrum.00472-21. Epub 2021 Aug 11. Microbiol Spectr. 2021. PMID: 34378968 Free PMC article.
-
SARS-CoV-2 replication and drug discovery.Mol Cell Probes. 2024 Oct;77:101973. doi: 10.1016/j.mcp.2024.101973. Epub 2024 Jul 24. Mol Cell Probes. 2024. PMID: 39025272 Review.
Cited by
-
SARS-CoV-2 genetic diversity in Venezuela: Predominance of D614G variants and analysis of one outbreak.PLoS One. 2021 Feb 19;16(2):e0247196. doi: 10.1371/journal.pone.0247196. eCollection 2021. PLoS One. 2021. PMID: 33606828 Free PMC article.
-
SARS- CoV-2 infection and oxidative stress in early-onset preeclampsia.Biochim Biophys Acta Mol Basis Dis. 2022 Mar 1;1868(3):166321. doi: 10.1016/j.bbadis.2021.166321. Epub 2021 Dec 14. Biochim Biophys Acta Mol Basis Dis. 2022. PMID: 34920081 Free PMC article. Review.
-
Accelerating drug repurposing for COVID-19 treatment by modeling mechanisms of action using cell image features and machine learning.Cogn Neurodyn. 2023 Jun;17(3):803-811. doi: 10.1007/s11571-021-09727-5. Epub 2021 Nov 5. Cogn Neurodyn. 2023. PMID: 34777628 Free PMC article.
-
Neutralization of Different Variants of SARS-CoV-2 by a F(ab')2 Preparation from Sera of Horses Immunized with the Viral Receptor Binding Domain.Antibodies (Basel). 2023 Dec 7;12(4):80. doi: 10.3390/antib12040080. Antibodies (Basel). 2023. PMID: 38131802 Free PMC article.
-
Effectiveness of a multidrug therapy consisting of Ivermectin, Azithromycin, Montelukast, and Acetylsalicylic acid to prevent hospitalization and death among ambulatory COVID-19 cases in Tlaxcala, Mexico.Int J Infect Dis. 2021 Apr;105:598-605. doi: 10.1016/j.ijid.2021.02.014. Epub 2021 Feb 10. Int J Infect Dis. 2021. PMID: 33578014 Free PMC article.
References
-
- Sanders JM, Monogue ML, Jodlowski TZ, Cutrell JB. Pharmacologic Treatments for Coronavirus Disease 2019 (COVID-19): A Review. JAMA. 2020 Apr;••• - PubMed
Publication types
MeSH terms
Substances
LinkOut - more resources
Full Text Sources
Other Literature Sources
Miscellaneous