Selective neuronal degeneration in MATR3 S85C knock-in mouse model of early-stage ALS
- PMID: 33082323
- PMCID: PMC7576598
- DOI: 10.1038/s41467-020-18949-w
Selective neuronal degeneration in MATR3 S85C knock-in mouse model of early-stage ALS
Abstract
A missense mutation, S85C, in the MATR3 gene is a genetic cause for amyotrophic lateral sclerosis (ALS). It is unclear how the S85C mutation affects MATR3 function and contributes to disease. Here, we develop a mouse model that harbors the S85C mutation in the endogenous Matr3 locus using the CRISPR/Cas9 system. MATR3 S85C knock-in mice recapitulate behavioral and neuropathological features of early-stage ALS including motor impairment, muscle atrophy, neuromuscular junction defects, Purkinje cell degeneration and neuroinflammation in the cerebellum and spinal cord. Our neuropathology data reveals a loss of MATR3 S85C protein in the cell bodies of Purkinje cells and motor neurons, suggesting that a decrease in functional MATR3 levels or loss of MATR3 function contributes to neuronal defects. Our findings demonstrate that the MATR3 S85C mouse model mimics aspects of early-stage ALS and would be a promising tool for future basic and preclinical research.
Conflict of interest statement
The authors declare no competing interests.
Figures
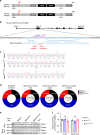
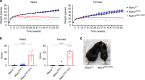
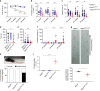
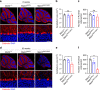
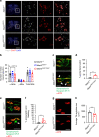
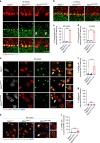
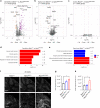
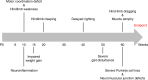
Similar articles
-
Selective Loss of MATR3 in Spinal Interneurons, Upper Motor Neurons and Hippocampal CA1 Neurons in a MATR3 S85C Knock-In Mouse Model of Amyotrophic Lateral Sclerosis.Biology (Basel). 2022 Feb 12;11(2):298. doi: 10.3390/biology11020298. Biology (Basel). 2022. PMID: 35205163 Free PMC article.
-
A mutant MATR3 mouse model to explain multisystem proteinopathy.J Pathol. 2019 Oct;249(2):182-192. doi: 10.1002/path.5289. Epub 2019 Jun 18. J Pathol. 2019. PMID: 31056746
-
MATR3 F115C knock-in mice do not exhibit motor defects or neuropathological features of ALS.Biochem Biophys Res Commun. 2021 Sep 3;568:48-54. doi: 10.1016/j.bbrc.2021.06.052. Epub 2021 Jun 26. Biochem Biophys Res Commun. 2021. PMID: 34182213
-
MATR3's Role beyond the Nuclear Matrix: From Gene Regulation to Its Implications in Amyotrophic Lateral Sclerosis and Other Diseases.Cells. 2024 Jun 5;13(11):980. doi: 10.3390/cells13110980. Cells. 2024. PMID: 38891112 Free PMC article. Review.
-
Matrin 3 in neuromuscular disease: physiology and pathophysiology.JCI Insight. 2021 Jan 11;6(1):e143948. doi: 10.1172/jci.insight.143948. JCI Insight. 2021. PMID: 33427209 Free PMC article. Review.
Cited by
-
Amyotrophic Lateral Sclerosis: Insights and New Prospects in Disease Pathophysiology, Biomarkers and Therapies.Pharmaceuticals (Basel). 2024 Oct 18;17(10):1391. doi: 10.3390/ph17101391. Pharmaceuticals (Basel). 2024. PMID: 39459030 Free PMC article. Review.
-
Knockout of Dectin-1 does not modify disease onset or progression in a MATR3 S85C knock-in mouse model of ALS.Heliyon. 2024 Sep 14;10(18):e37926. doi: 10.1016/j.heliyon.2024.e37926. eCollection 2024 Sep 30. Heliyon. 2024. PMID: 39323783 Free PMC article.
-
Hepatocellular carcinoma-specific epigenetic checkpoints bidirectionally regulate the antitumor immunity of CD4 + T cells.Cell Mol Immunol. 2024 Nov;21(11):1296-1308. doi: 10.1038/s41423-024-01215-0. Epub 2024 Sep 19. Cell Mol Immunol. 2024. PMID: 39300319
-
The role of Matrin-3 in physiology and its dysregulation in disease.Biochem Soc Trans. 2024 Jun 26;52(3):961-972. doi: 10.1042/BST20220585. Biochem Soc Trans. 2024. PMID: 38813817 Free PMC article. Review.
-
Executive Summary of 2023 International Conference of the Korean Dementia Association (IC-KDA 2023): A Report From the Academic Committee of the Korean Dementia Association.Dement Neurocogn Disord. 2024 Apr;23(2):75-88. doi: 10.12779/dnd.2024.23.2.75. Epub 2024 Apr 24. Dement Neurocogn Disord. 2024. PMID: 38720824 Free PMC article. Review.
References
Publication types
MeSH terms
Substances
Associated data
LinkOut - more resources
Full Text Sources
Medical
Molecular Biology Databases
Miscellaneous