Cancer-associated mutations in the condensin II subunit CAPH2 cause genomic instability through telomere dysfunction and anaphase chromosome bridges
- PMID: 33078399
- PMCID: PMC7983937
- DOI: 10.1002/jcp.30113
Cancer-associated mutations in the condensin II subunit CAPH2 cause genomic instability through telomere dysfunction and anaphase chromosome bridges
Abstract
Genome instability in cancer drives tumor heterogeneity, undermines the success of therapies, and leads to metastasis and recurrence. Condensins are conserved chromatin-binding proteins that promote genomic stability, mainly by ensuring proper condensation of chromatin and mitotic chromosome segregation. Condensin mutations are found in human tumors, but it is not known how or even if such mutations promote cancer progression. In this study, we focus on condensin II subunit CAPH2 and specific CAPH2 mutations reported to be enriched in human cancer patients, and we test how CAPH2 cancer-specific mutations may lead to condensin II complex dysfunction and contribute to genome instability. We find that R551P, R551S, and S556F mutations in CAPH2 cause genomic instability by causing DNA damage, anaphase defects, micronuclei, and chromosomal instability. DNA damage and anaphase defects are caused primarily by ataxia telangiectasia and Rad3-related-dependent telomere dysfunction, as anaphase bridges are enriched for telomeric repeat sequences. We also show that these mutations decrease the binding of CAPH2 to the ATPase subunit SMC4 as well as the rest of the condensin II complex, and decrease the amount of CAPH2 protein bound to chromatin. Thus, in vivo the R551P, R551S, and S556F cancer-specific CAPH2 mutant proteins are likely to impair condensin II complex formation, impede condensin II activity during mitosis and interphase, and promote genetic heterogeneity in cell populations that can lead to clonal outgrowth of cancer cells with highly diverse genotypes.
Keywords: DNA damage; anaphase bridge; condensin; genome instability; telomere.
© 2020 The Authors. Journal of Cellular Physiology published by Wiley Periodicals LLC.
Conflict of interest statement
The authors declare that there are no conflicts of interests.
Figures
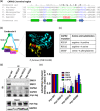
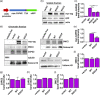
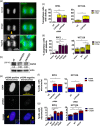
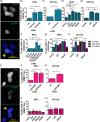
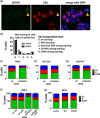
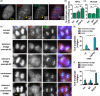
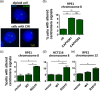
Similar articles
-
Condensin II mutation causes T-cell lymphoma through tissue-specific genome instability.Genes Dev. 2016 Oct 1;30(19):2173-2186. doi: 10.1101/gad.284562.116. Epub 2016 Oct 13. Genes Dev. 2016. PMID: 27737961 Free PMC article.
-
Condensin II resolves chromosomal associations to enable anaphase I segregation in Drosophila male meiosis.PLoS Genet. 2008 Oct;4(10):e1000228. doi: 10.1371/journal.pgen.1000228. Epub 2008 Oct 17. PLoS Genet. 2008. PMID: 18927632 Free PMC article.
-
Condensin II subunit NCAPH2 associates with shelterin protein TRF1 and is required for telomere stability.J Cell Physiol. 2019 Nov;234(11):20755-20768. doi: 10.1002/jcp.28681. Epub 2019 Apr 26. J Cell Physiol. 2019. PMID: 31026066 Free PMC article.
-
The structure and function of SMC and kleisin complexes.Annu Rev Biochem. 2005;74:595-648. doi: 10.1146/annurev.biochem.74.082803.133219. Annu Rev Biochem. 2005. PMID: 15952899 Review.
-
Condensins and cohesins - one of these things is not like the other!J Cell Sci. 2019 Feb 7;132(3):jcs220491. doi: 10.1242/jcs.220491. J Cell Sci. 2019. PMID: 30733374 Free PMC article. Review.
Cited by
-
NCAPH Drives Breast Cancer Progression and Identifies a Gene Signature that Predicts Luminal A Tumor Recurrence.Res Sq [Preprint]. 2023 Oct 16:rs.3.rs-3231230. doi: 10.21203/rs.3.rs-3231230/v2. Res Sq. 2023. Update in: Clin Transl Med. 2024 Feb;14(2):e1554. doi: 10.1002/ctm2.1554. PMID: 37886490 Free PMC article. Updated. Preprint.
-
Epigenetic instability caused by absence of CIZ1 drives transformation during quiescence cycles.BMC Biol. 2023 Aug 15;21(1):175. doi: 10.1186/s12915-023-01671-6. BMC Biol. 2023. PMID: 37580709 Free PMC article.
-
A Somatic Mutation-Derived LncRNA Signature of Genomic Instability Predicts Prognosis for Patients With Liver Cancer.Front Surg. 2021 Aug 24;8:724792. doi: 10.3389/fsurg.2021.724792. eCollection 2021. Front Surg. 2021. PMID: 34504866 Free PMC article.
-
Multilevel view on chromatin architecture alterations in cancer.Front Genet. 2022 Nov 18;13:1059617. doi: 10.3389/fgene.2022.1059617. eCollection 2022. Front Genet. 2022. PMID: 36468037 Free PMC article. Review.
-
The footprint of gut microbiota in gallbladder cancer: a mechanistic review.Front Cell Infect Microbiol. 2024 May 7;14:1374238. doi: 10.3389/fcimb.2024.1374238. eCollection 2024. Front Cell Infect Microbiol. 2024. PMID: 38774627 Free PMC article. Review.
References
-
- d'Adda di Fagagna, Reaper, P. M. , Clay‐Farrace, L. , Fiegler, H. , Carr, P. , Von Zglinicki, T. , Saretzki, G. , Carter, N. P. , & Jackson, S. P. (2003). A DNA damage checkpoint response in telomere‐initiated senescence. Nature, 426, 194–198. - PubMed
Publication types
MeSH terms
Substances
Grants and funding
LinkOut - more resources
Full Text Sources
Molecular Biology Databases
Research Materials