Expansion of Intrinsically Disordered Proteins Increases the Range of Stability of Liquid-Liquid Phase Separation
- PMID: 33076213
- PMCID: PMC7587599
- DOI: 10.3390/molecules25204705
Expansion of Intrinsically Disordered Proteins Increases the Range of Stability of Liquid-Liquid Phase Separation
Abstract
Proteins containing intrinsically disordered regions (IDRs) are ubiquitous within biomolecular condensates, which are liquid-like compartments within cells formed through liquid-liquid phase separation (LLPS). The sequence of amino acids of a protein encodes its phase behaviour, not only by establishing the patterning and chemical nature (e.g., hydrophobic, polar, charged) of the various binding sites that facilitate multivalent interactions, but also by dictating the protein conformational dynamics. Besides behaving as random coils, IDRs can exhibit a wide-range of structural behaviours, including conformational switching, where they transition between alternate conformational ensembles. Using Molecular Dynamics simulations of a minimal coarse-grained model for IDRs, we show that the role of protein conformation has a non-trivial effect in the liquid-liquid phase behaviour of IDRs. When an IDR transitions to a conformational ensemble enriched in disordered extended states, LLPS is enhanced. In contrast, IDRs that switch to ensembles that preferentially sample more compact and structured states show inhibited LLPS. This occurs because extended and disordered protein conformations facilitate LLPS-stabilising multivalent protein-protein interactions by reducing steric hindrance; thereby, such conformations maximize the molecular connectivity of the condensed liquid network. Extended protein configurations promote phase separation regardless of whether LLPS is driven by homotypic and/or heterotypic protein-protein interactions. This study sheds light on the link between the dynamic conformational plasticity of IDRs and their liquid-liquid phase behaviour.
Keywords: biological phase transitions; computer simulations; proteins.
Conflict of interest statement
The authors declare no conflict of interest.
Figures
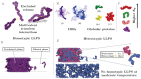
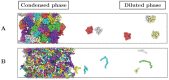
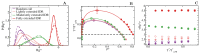
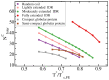
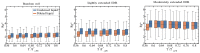
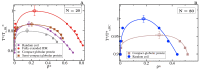
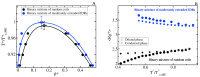
Similar articles
-
Classification of proteins inducing liquid-liquid phase separation: sequential, structural and functional characterization.J Biochem. 2023 Mar 31;173(4):255-264. doi: 10.1093/jb/mvac106. J Biochem. 2023. PMID: 36575582 Free PMC article.
-
Liquid-Liquid Phase Separation by Intrinsically Disordered Protein Regions of Viruses: Roles in Viral Life Cycle and Control of Virus-Host Interactions.Int J Mol Sci. 2020 Nov 28;21(23):9045. doi: 10.3390/ijms21239045. Int J Mol Sci. 2020. PMID: 33260713 Free PMC article. Review.
-
Targeted modulation of protein liquid-liquid phase separation by evolution of amino-acid sequence.PLoS Comput Biol. 2021 Aug 24;17(8):e1009328. doi: 10.1371/journal.pcbi.1009328. eCollection 2021 Aug. PLoS Comput Biol. 2021. PMID: 34428231 Free PMC article.
-
A call to order: Examining structured domains in biomolecular condensates.J Magn Reson. 2023 Jan;346:107318. doi: 10.1016/j.jmr.2022.107318. J Magn Reson. 2023. PMID: 36657879 Free PMC article.
-
Towards Decoding the Sequence-Based Grammar Governing the Functions of Intrinsically Disordered Protein Regions.J Mol Biol. 2021 Jun 11;433(12):166724. doi: 10.1016/j.jmb.2020.11.023. Epub 2020 Nov 26. J Mol Biol. 2021. PMID: 33248138 Review.
Cited by
-
Toward Accurate Simulation of Coupling between Protein Secondary Structure and Phase Separation.J Am Chem Soc. 2024 Jan 10;146(1):342-357. doi: 10.1021/jacs.3c09195. Epub 2023 Dec 19. J Am Chem Soc. 2024. PMID: 38112495
-
SMA-linked SMN mutants prevent phase separation properties and SMN interactions with FMRP family members.Life Sci Alliance. 2022 Nov 14;6(1):e202201429. doi: 10.26508/lsa.202201429. Print 2023 Jan. Life Sci Alliance. 2022. PMID: 36375840 Free PMC article.
-
RNA length has a non-trivial effect in the stability of biomolecular condensates formed by RNA-binding proteins.PLoS Comput Biol. 2022 Feb 2;18(2):e1009810. doi: 10.1371/journal.pcbi.1009810. eCollection 2022 Feb. PLoS Comput Biol. 2022. PMID: 35108264 Free PMC article.
-
Myofilament-associated proteins with intrinsic disorder (MAPIDs) and their resolution by computational modeling.Q Rev Biophys. 2023 Jan 11;56:e2. doi: 10.1017/S003358352300001X. Q Rev Biophys. 2023. PMID: 36628457 Free PMC article. Review.
-
Surfactants or scaffolds? RNAs of varying lengths control the thermodynamic stability of condensates differently.Biophys J. 2023 Jul 25;122(14):2973-2987. doi: 10.1016/j.bpj.2023.03.006. Epub 2023 Mar 6. Biophys J. 2023. PMID: 36883003 Free PMC article.
References
MeSH terms
Substances
Grants and funding
LinkOut - more resources
Full Text Sources