Controlling emerging zoonoses at the animal-human interface
- PMID: 33073176
- PMCID: PMC7550773
- DOI: 10.1186/s42522-020-00024-5
Controlling emerging zoonoses at the animal-human interface
Abstract
Background: For many emerging or re-emerging pathogens, cases in humans arise from a mixture of introductions (via zoonotic spillover from animal reservoirs or geographic spillover from endemic regions) and secondary human-to-human transmission. Interventions aiming to reduce incidence of these infections can be focused on preventing spillover or reducing human-to-human transmission, or sometimes both at once, and typically are governed by resource constraints that require policymakers to make choices. Despite increasing emphasis on using mathematical models to inform disease control policies, little attention has been paid to guiding rational disease control at the animal-human interface.
Methods: We introduce a modeling framework to analyze the impacts of different disease control policies, focusing on pathogens exhibiting subcritical transmission among humans (i.e. pathogens that cannot establish sustained human-to-human transmission). We quantify the relative effectiveness of measures to reduce spillover (e.g. reducing contact with animal hosts), human-to-human transmission (e.g. case isolation), or both at once (e.g. vaccination), across a range of epidemiological contexts.
Results: We provide guidelines for choosing which mode of control to prioritize in different epidemiological scenarios and considering different levels of resource and relative costs. We contextualize our analysis with current zoonotic pathogens and other subcritical pathogens, such as post-elimination measles, and control policies that have been applied.
Conclusions: Our work provides a model-based, theoretical foundation to understand and guide policy for subcritical zoonoses, integrating across disciplinary and species boundaries in a manner consistent with One Health principles.
Keywords: Cross-species spillover transmission; Emerging infectious diseases; Epidemiological control; Human-to-human transmission; Infectious disease dynamics; Stuttering zoonoses; Subcritical zoonoses.
© The Author(s) 2020.
Conflict of interest statement
Competing interestsThe authors declare no competing interests.
Figures
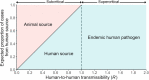
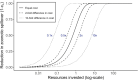
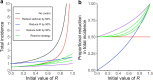
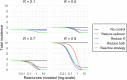
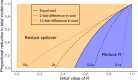
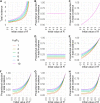
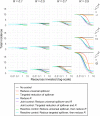
Similar articles
-
Modeling spillover dynamics: understanding emerging pathogens of public health concern.Sci Rep. 2024 Apr 29;14(1):9823. doi: 10.1038/s41598-024-60661-y. Sci Rep. 2024. PMID: 38684927 Free PMC article.
-
Policies to prevent zoonotic spillover: a systematic scoping review of evaluative evidence.Global Health. 2023 Nov 8;19(1):82. doi: 10.1186/s12992-023-00986-x. Global Health. 2023. PMID: 37940941 Free PMC article. Review.
-
Stochastic dynamics of an epidemic with recurrent spillovers from an endemic reservoir.J Theor Biol. 2018 Nov 14;457:37-50. doi: 10.1016/j.jtbi.2018.08.017. Epub 2018 Aug 16. J Theor Biol. 2018. PMID: 30121292 Free PMC article.
-
A Unified Framework for the Infection Dynamics of Zoonotic Spillover and Spread.PLoS Negl Trop Dis. 2016 Sep 2;10(9):e0004957. doi: 10.1371/journal.pntd.0004957. eCollection 2016 Sep. PLoS Negl Trop Dis. 2016. PMID: 27588425 Free PMC article.
-
Pathways to zoonotic spillover.Nat Rev Microbiol. 2017 Aug;15(8):502-510. doi: 10.1038/nrmicro.2017.45. Epub 2017 May 30. Nat Rev Microbiol. 2017. PMID: 28555073 Free PMC article. Review.
Cited by
-
Mathematical modeling of mpox: A scoping review.One Health. 2023 Jun;16:100540. doi: 10.1016/j.onehlt.2023.100540. Epub 2023 Apr 17. One Health. 2023. PMID: 37138928 Free PMC article. Review.
-
Host-Pathogen Interactions Influencing Zoonotic Spillover Potential and Transmission in Humans.Viruses. 2023 Feb 22;15(3):599. doi: 10.3390/v15030599. Viruses. 2023. PMID: 36992308 Free PMC article. Review.
References
-
- McMahon BJ, Morand S, Gray JS. Ecosystem change and zoonoses in the Anthropocene. Zoonoses Public Health. 2018;65(7):755–765. - PubMed