PML nuclear bodies and chromatin dynamics: catch me if you can!
- PMID: 33068409
- PMCID: PMC7708061
- DOI: 10.1093/nar/gkaa828
PML nuclear bodies and chromatin dynamics: catch me if you can!
Abstract
Eukaryotic cells compartmentalize their internal milieu in order to achieve specific reactions in time and space. This organization in distinct compartments is essential to allow subcellular processing of regulatory signals and generate specific cellular responses. In the nucleus, genetic information is packaged in the form of chromatin, an organized and repeated nucleoprotein structure that is a source of epigenetic information. In addition, cells organize the distribution of macromolecules via various membrane-less nuclear organelles, which have gathered considerable attention in the last few years. The macromolecular multiprotein complexes known as Promyelocytic Leukemia Nuclear Bodies (PML NBs) are an archetype for nuclear membrane-less organelles. Chromatin interactions with nuclear bodies are important to regulate genome function. In this review, we will focus on the dynamic interplay between PML NBs and chromatin. We report how the structure and formation of PML NBs, which may involve phase separation mechanisms, might impact their functions in the regulation of chromatin dynamics. In particular, we will discuss how PML NBs participate in the chromatinization of viral genomes, as well as in the control of specific cellular chromatin assembly pathways which govern physiological mechanisms such as senescence or telomere maintenance.
© The Author(s) 2020. Published by Oxford University Press on behalf of Nucleic Acids Research.
Figures
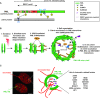
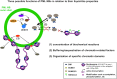
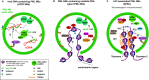
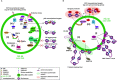
Similar articles
-
Promyelocytic leukemia (PML) nuclear bodies (NBs) induce latent/quiescent HSV-1 genomes chromatinization through a PML NB/Histone H3.3/H3.3 Chaperone Axis.PLoS Pathog. 2018 Sep 20;14(9):e1007313. doi: 10.1371/journal.ppat.1007313. eCollection 2018 Sep. PLoS Pathog. 2018. PMID: 30235352 Free PMC article.
-
Swine Promyelocytic Leukemia Isoform II Inhibits Pseudorabies Virus Infection by Suppressing Viral Gene Transcription in Promyelocytic Leukemia Nuclear Bodies.J Virol. 2020 Aug 31;94(18):e01197-20. doi: 10.1128/JVI.01197-20. Print 2020 Aug 31. J Virol. 2020. PMID: 32641476 Free PMC article.
-
Nuclear bodies and compartments: functional roles and cellular signalling in health and disease.Cell Signal. 2004 Oct;16(10):1085-104. doi: 10.1016/j.cellsig.2004.03.020. Cell Signal. 2004. PMID: 15240004 Review.
-
SUMO5, a Novel Poly-SUMO Isoform, Regulates PML Nuclear Bodies.Sci Rep. 2016 May 23;6:26509. doi: 10.1038/srep26509. Sci Rep. 2016. PMID: 27211601 Free PMC article.
-
SUMOrganization of the nucleus.Curr Opin Cell Biol. 2007 Jun;19(3):350-5. doi: 10.1016/j.ceb.2007.04.014. Epub 2007 Apr 27. Curr Opin Cell Biol. 2007. PMID: 17467254 Review.
Cited by
-
TDP-43 and NEAT long non-coding RNA: Roles in neurodegenerative disease.Front Cell Neurosci. 2022 Oct 26;16:954912. doi: 10.3389/fncel.2022.954912. eCollection 2022. Front Cell Neurosci. 2022. PMID: 36385948 Free PMC article. Review.
-
Immune chromatin reader SP140 regulates microbiota and risk for inflammatory bowel disease.Cell Host Microbe. 2022 Oct 12;30(10):1370-1381.e5. doi: 10.1016/j.chom.2022.08.018. Epub 2022 Sep 20. Cell Host Microbe. 2022. PMID: 36130593 Free PMC article.
-
Nuclear structures and their emerging roles in cell differentiation and development.BMB Rep. 2024 Sep;57(9):381-387. doi: 10.5483/BMBRep.2024-0101. BMB Rep. 2024. PMID: 39219044 Free PMC article. Review.
-
Differential contributions of phosphotransferases CEPT1 and CHPT1 to phosphatidylcholine homeostasis and lipid droplet biogenesis.J Biol Chem. 2023 Apr;299(4):104578. doi: 10.1016/j.jbc.2023.104578. Epub 2023 Mar 3. J Biol Chem. 2023. PMID: 36871755 Free PMC article.
-
Nuclear Compartments: An Incomplete Primer to Nuclear Compartments, Bodies, and Genome Organization Relative to Nuclear Architecture.Cold Spring Harb Perspect Biol. 2022 Jul 1;14(7):a041268. doi: 10.1101/cshperspect.a041268. Cold Spring Harb Perspect Biol. 2022. PMID: 34400557 Free PMC article. Review.
References
Publication types
MeSH terms
Substances
LinkOut - more resources
Full Text Sources
Research Materials
Miscellaneous