Synapse type-specific proteomic dissection identifies IgSF8 as a hippocampal CA3 microcircuit organizer
- PMID: 33057002
- PMCID: PMC7560607
- DOI: 10.1038/s41467-020-18956-x
Synapse type-specific proteomic dissection identifies IgSF8 as a hippocampal CA3 microcircuit organizer
Abstract
Excitatory and inhibitory neurons are connected into microcircuits that generate circuit output. Central in the hippocampal CA3 microcircuit is the mossy fiber (MF) synapse, which provides powerful direct excitatory input and indirect feedforward inhibition to CA3 pyramidal neurons. Here, we dissect its cell-surface protein (CSP) composition to discover novel regulators of MF synaptic connectivity. Proteomic profiling of isolated MF synaptosomes uncovers a rich CSP composition, including many CSPs without synaptic function and several that are uncharacterized. Cell-surface interactome screening identifies IgSF8 as a neuronal receptor enriched in the MF pathway. Presynaptic Igsf8 deletion impairs MF synaptic architecture and robustly decreases the density of bouton filopodia that provide feedforward inhibition. Consequently, IgSF8 loss impairs excitation/inhibition balance and increases excitability of CA3 pyramidal neurons. Our results provide insight into the CSP landscape and interactome of a specific excitatory synapse and reveal IgSF8 as a critical regulator of CA3 microcircuit connectivity and function.
Conflict of interest statement
The authors declare no competing interests.
Figures
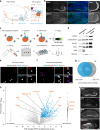
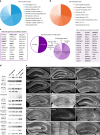
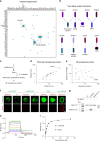
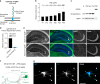
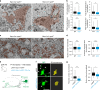
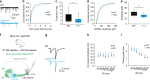
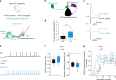
Similar articles
-
Intracellular Zn2+ Signaling Facilitates Mossy Fiber Input-Induced Heterosynaptic Potentiation of Direct Cortical Inputs in Hippocampal CA3 Pyramidal Cells.J Neurosci. 2019 May 15;39(20):3812-3831. doi: 10.1523/JNEUROSCI.2130-18.2019. Epub 2019 Mar 4. J Neurosci. 2019. PMID: 30833508 Free PMC article.
-
Single Bursts of Individual Granule Cells Functionally Rearrange Feedforward Inhibition.J Neurosci. 2018 Feb 14;38(7):1711-1724. doi: 10.1523/JNEUROSCI.1595-17.2018. Epub 2018 Jan 15. J Neurosci. 2018. PMID: 29335356 Free PMC article.
-
Hippocampal Mossy Fibers Synapses in CA3 Pyramidal Cells Are Altered at an Early Stage in a Mouse Model of Alzheimer's Disease.J Neurosci. 2019 May 22;39(21):4193-4205. doi: 10.1523/JNEUROSCI.2868-18.2019. Epub 2019 Mar 18. J Neurosci. 2019. PMID: 30886015 Free PMC article.
-
Hippocampal mossy fiber synaptic transmission and its modulation.Vitam Horm. 2010;82:65-85. doi: 10.1016/S0083-6729(10)82004-7. Vitam Horm. 2010. PMID: 20472133 Review.
-
BDNF and Lactate as Modulators of Hippocampal CA3 Network Physiology.Cell Mol Neurobiol. 2023 Nov;43(8):4007-4022. doi: 10.1007/s10571-023-01425-6. Epub 2023 Oct 24. Cell Mol Neurobiol. 2023. PMID: 37874456 Review.
Cited by
-
Non-canonical function of ADAM10 in presynaptic plasticity.Cell Mol Life Sci. 2024 Aug 9;81(1):342. doi: 10.1007/s00018-024-05327-8. Cell Mol Life Sci. 2024. PMID: 39123091 Free PMC article.
-
Postnatal persistence of hippocampal Cajal-Retzius cells has a crucial role in the establishment of the hippocampal circuit.Development. 2024 Jan 1;151(1):dev202236. doi: 10.1242/dev.202236. Epub 2024 Jan 9. Development. 2024. PMID: 38095282 Free PMC article.
-
Optogenetic inhibition of the dorsal hippocampus CA3 region during early-stage cocaine-memory reconsolidation disrupts subsequent context-induced cocaine seeking in rats.Neuropsychopharmacology. 2022 Jul;47(8):1473-1483. doi: 10.1038/s41386-022-01342-0. Epub 2022 May 17. Neuropsychopharmacology. 2022. PMID: 35581381 Free PMC article.
-
LRRTM3 regulates activity-dependent synchronization of synapse properties in topographically connected hippocampal neural circuits.Proc Natl Acad Sci U S A. 2022 Jan 18;119(3):e2110196119. doi: 10.1073/pnas.2110196119. Proc Natl Acad Sci U S A. 2022. PMID: 35022233 Free PMC article.
-
Synaptic recognition molecules in development and disease.Curr Top Dev Biol. 2021;142:319-370. doi: 10.1016/bs.ctdb.2020.12.009. Epub 2021 Feb 12. Curr Top Dev Biol. 2021. PMID: 33706921 Free PMC article.
References
Publication types
MeSH terms
Substances
Grants and funding
LinkOut - more resources
Full Text Sources
Other Literature Sources
Molecular Biology Databases
Research Materials
Miscellaneous