Phase-Separated Transcriptional Condensates Accelerate Target-Search Process Revealed by Live-Cell Single-Molecule Imaging
- PMID: 33053359
- PMCID: PMC7593837
- DOI: 10.1016/j.celrep.2020.108248
Phase-Separated Transcriptional Condensates Accelerate Target-Search Process Revealed by Live-Cell Single-Molecule Imaging
Abstract
Compartmentalization by liquid-liquid phase separation is implicated in transcription. It remains unclear whether and how transcriptional condensates accelerate the search of transcriptional regulatory factors for their target sites. Furthermore, the molecular mechanisms by which regulatory factors nucleate on chromatin to assemble transcriptional condensates remain incompletely understood. The CBX-PRC1 complexes compartmentalize key developmental regulators for repression through phase-separated condensates driven by the chromobox 2 (CBX2) protein. Here, by using live-cell single-molecule imaging, we show that CBX2 nucleates on chromatin independently of H3K27me3 and CBX-PRC1. The interactions between CBX2 and DNA are essential for nucleating CBX-PRC1 on chromatin to assemble condensates. The assembled condensates shorten 3D diffusion time and reduce trials for finding specific sites through revisiting the same or adjacent sites repetitively, thereby accelerating CBX2 in searching for target sites. Overall, our data suggest a generic mechanism by which transcriptional regulatory factors nucleate to assemble condensates that accelerate their target-search process.
Keywords: CBX2; PRC1; PcG; chromatin; compartmentalization; epigenetics; liquid-liquid phase separation; nucleation; single-molecule imaging; target-search kinetics.
Copyright © 2020 The Authors. Published by Elsevier Inc. All rights reserved.
Conflict of interest statement
Declaration of Interests The authors declare no competing interests.
Figures
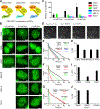
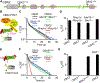
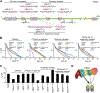
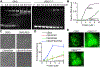
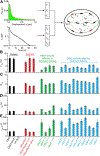
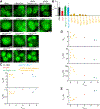
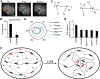
Similar articles
-
Nuclear condensates of the Polycomb protein chromobox 2 (CBX2) assemble through phase separation.J Biol Chem. 2019 Feb 1;294(5):1451-1463. doi: 10.1074/jbc.RA118.006620. Epub 2018 Dec 4. J Biol Chem. 2019. PMID: 30514760 Free PMC article.
-
Principles of assembly and regulation of condensates of Polycomb repressive complex 1 through phase separation.Cell Rep. 2023 Oct 31;42(10):113136. doi: 10.1016/j.celrep.2023.113136. Epub 2023 Sep 26. Cell Rep. 2023. PMID: 37756159 Free PMC article.
-
Live-cell single-molecule tracking reveals co-recognition of H3K27me3 and DNA targets polycomb Cbx7-PRC1 to chromatin.Elife. 2016 Oct 10;5:e17667. doi: 10.7554/eLife.17667. Elife. 2016. PMID: 27723458 Free PMC article.
-
Single-molecule imaging of epigenetic complexes in living cells: insights from studies on Polycomb group proteins.Nucleic Acids Res. 2021 Jul 9;49(12):6621-6637. doi: 10.1093/nar/gkab304. Nucleic Acids Res. 2021. PMID: 34009336 Free PMC article. Review.
-
Epigenetic regulation by polycomb group complexes: focus on roles of CBX proteins.J Zhejiang Univ Sci B. 2014 May;15(5):412-28. doi: 10.1631/jzus.B1400077. J Zhejiang Univ Sci B. 2014. PMID: 24793759 Free PMC article. Review.
Cited by
-
Mammalian PRC1 Complexes: Compositional Complexity and Diverse Molecular Mechanisms.Int J Mol Sci. 2020 Nov 14;21(22):8594. doi: 10.3390/ijms21228594. Int J Mol Sci. 2020. PMID: 33202645 Free PMC article. Review.
-
How a disordered linker in the Polycomb protein Polyhomeotic tunes phase separation and oligomerization.bioRxiv [Preprint]. 2023 Oct 27:2023.10.26.564264. doi: 10.1101/2023.10.26.564264. bioRxiv. 2023. PMID: 37961422 Free PMC article. Preprint.
-
The needle and the haystack: single molecule tracking to probe the transcription factor search in eukaryotes.Biochem Soc Trans. 2021 Jun 30;49(3):1121-1132. doi: 10.1042/BST20200709. Biochem Soc Trans. 2021. PMID: 34003257 Free PMC article. Review.
-
Liquid-Liquid Phase Separation in Chromatin.Cold Spring Harb Perspect Biol. 2022 Feb 1;14(2):a040683. doi: 10.1101/cshperspect.a040683. Cold Spring Harb Perspect Biol. 2022. PMID: 34127447 Free PMC article. Review.
-
Explicit ion modeling predicts physicochemical interactions for chromatin organization.Elife. 2024 Jan 30;12:RP90073. doi: 10.7554/eLife.90073. Elife. 2024. PMID: 38289342 Free PMC article.
References
Publication types
MeSH terms
Substances
Grants and funding
LinkOut - more resources
Full Text Sources