Myocardial Energy Metabolism in Non-ischemic Cardiomyopathy
- PMID: 33041869
- PMCID: PMC7526697
- DOI: 10.3389/fphys.2020.570421
Myocardial Energy Metabolism in Non-ischemic Cardiomyopathy
Abstract
As the most metabolically demanding organ in the body, the heart must generate massive amounts of energy adenosine triphosphate (ATP) from the oxidation of fatty acids, carbohydrates and other fuels (e.g., amino acids, ketone bodies), in order to sustain constant contractile function. While the healthy mature heart acts omnivorously and is highly flexible in its ability to utilize the numerous fuel sources delivered to it through its coronary circulation, the heart's ability to produce ATP from these fuel sources becomes perturbed in numerous cardiovascular disorders. This includes ischemic heart disease and myocardial infarction, as well as in various cardiomyopathies that often precede the development of overt heart failure. We herein will provide an overview of myocardial energy metabolism in the healthy heart, while describing the numerous perturbations that take place in various non-ischemic cardiomyopathies such as hypertrophic cardiomyopathy, diabetic cardiomyopathy, arrhythmogenic cardiomyopathy, and the cardiomyopathy associated with the rare genetic disease, Barth Syndrome. Based on preclinical evidence where optimizing myocardial energy metabolism has been shown to attenuate cardiac dysfunction, we will discuss the feasibility of myocardial energetics optimization as an approach to treat the cardiac pathology associated with these various non-ischemic cardiomyopathies.
Keywords: cardiac function; cardiomyopathy; energy metabolism; fatty acid oxidation; glucose oxidation.
Copyright © 2020 Greenwell, Gopal and Ussher.
Figures
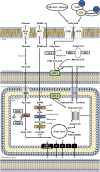
Similar articles
-
Myocardial disturbances of intermediary metabolism in Barth syndrome.Front Cardiovasc Med. 2022 Aug 10;9:981972. doi: 10.3389/fcvm.2022.981972. eCollection 2022. Front Cardiovasc Med. 2022. PMID: 36035919 Free PMC article. Review.
-
Alterations in energy metabolism in cardiomyopathies.Ann Med. 2007;39(8):594-607. doi: 10.1080/07853890701618305. Ann Med. 2007. PMID: 17934906 Review.
-
Heart Metabolism in Sepsis-Induced Cardiomyopathy-Unusual Metabolic Dysfunction of the Heart.Int J Environ Res Public Health. 2021 Jul 16;18(14):7598. doi: 10.3390/ijerph18147598. Int J Environ Res Public Health. 2021. PMID: 34300048 Free PMC article. Review.
-
Myocardial adenine nucleotide concentrations and myocardial norepinephrine content in patients with heart failure secondary to idiopathic dilated or ischemic cardiomyopathy.Am J Cardiol. 1992 Jun 15;69(19):1574-80. doi: 10.1016/0002-9149(92)90706-5. Am J Cardiol. 1992. PMID: 1598872
-
Effect of diabetes on alteration of metabolism in cardiac myocytes: therapeutic implications.Diabetes Technol Ther. 2011 Nov;13(11):1155-60. doi: 10.1089/dia.2011.0120. Epub 2011 Jul 13. Diabetes Technol Ther. 2011. PMID: 21751873 Review.
Cited by
-
Exercise Training after Myocardial Infarction Attenuates Dysfunctional Ventricular Remodeling and Promotes Cardiac Recovery.Rev Cardiovasc Med. 2022 Apr 19;23(4):148. doi: 10.31083/j.rcm2304148. eCollection 2022 Apr. Rev Cardiovasc Med. 2022. PMID: 39076229 Free PMC article. Review.
-
Targeting Energy Protection as a Novel Strategy to Disclose Di'ao Xinxuekang against the Cardiotoxicity Caused by Doxorubicin.Int J Mol Sci. 2023 Jan 4;24(2):897. doi: 10.3390/ijms24020897. Int J Mol Sci. 2023. PMID: 36674413 Free PMC article.
-
Disturbed Cardiac Metabolism Triggers Atrial Arrhythmogenesis in Diabetes Mellitus: Energy Substrate Alternate as a Potential Therapeutic Intervention.Cells. 2022 Sep 18;11(18):2915. doi: 10.3390/cells11182915. Cells. 2022. PMID: 36139490 Free PMC article. Review.
-
The effects of nicotinamide adenine dinucleotide in cardiovascular diseases: Molecular mechanisms, roles and therapeutic potential.Genes Dis. 2021 Apr 24;9(4):959-972. doi: 10.1016/j.gendis.2021.04.001. eCollection 2022 Jul. Genes Dis. 2021. PMID: 35685463 Free PMC article. Review.
-
Plasma homocysteine levels and risk of congestive heart failure or cardiomyopathy: A Mendelian randomization study.Front Cardiovasc Med. 2023 Jan 26;10:1030257. doi: 10.3389/fcvm.2023.1030257. eCollection 2023. Front Cardiovasc Med. 2023. PMID: 36776266 Free PMC article.
References
Publication types
LinkOut - more resources
Full Text Sources
Research Materials