First Insight into the Modulation of Noncanonical NF-κB Signaling Components by Poxviruses in Established Immune-Derived Cell Lines: An In Vitro Model of Ectromelia Virus Infection
- PMID: 33020446
- PMCID: PMC7599462
- DOI: 10.3390/pathogens9100814
First Insight into the Modulation of Noncanonical NF-κB Signaling Components by Poxviruses in Established Immune-Derived Cell Lines: An In Vitro Model of Ectromelia Virus Infection
Abstract
Dendritic cells (DCs) and macrophages are the first line of antiviral immunity. Viral pathogens exploit these cell populations for their efficient replication and dissemination via the modulation of intracellular signaling pathways. Disruption of the noncanonical nuclear factor κ-light-chain-enhancer of activated B cells (NF-κB) signaling has frequently been observed in lymphoid cells upon infection with oncogenic viruses. However, several nononcogenic viruses have been shown to manipulate the noncanonical NF-κB signaling in different cell types. This study demonstrates the modulating effect of ectromelia virus (ECTV) on the components of the noncanonical NF-κB signaling pathway in established murine cell lines: JAWS II DCs and RAW 264.7 macrophages. ECTV affected the activation of TRAF2, cIAP1, RelB, and p100 upon cell treatment with both canonical and noncanonical NF-κB stimuli and thus impeded DNA binding by RelB and p52. ECTV also inhibited the expression of numerous genes related to the noncanonical NF-κB pathway and RelB-dependent gene expression in the cells treated with canonical and noncanonical NF-κB activators. Thus, our data strongly suggest that ECTV influenced the noncanonical NF-κB signaling components in the in vitro models. These findings provide new insights into the noncanonical NF-κB signaling components and their manipulation by poxviruses in vitro.
Keywords: antiviral immunity; dendritic cells; ectromelia virus; macrophages; noncanonical NF-κB signaling.
Conflict of interest statement
The authors declare no conflict of interest. The funders had no role in the design of the study; in the collection, analyses, or interpretation of data; in the writing of the manuscript, or in the decision to publish the results.
Figures
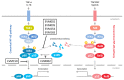
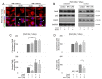
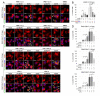
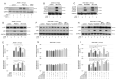
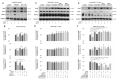
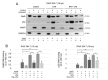
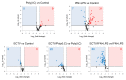
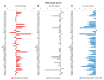
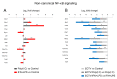
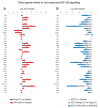
Similar articles
-
Modulation of proinflammatory NF-κB signaling by ectromelia virus in RAW 264.7 murine macrophages.Arch Virol. 2015 Sep;160(9):2301-14. doi: 10.1007/s00705-015-2507-y. Epub 2015 Jul 4. Arch Virol. 2015. PMID: 26141411
-
Late-phase synthesis of IκBα insulates the TLR4-activated canonical NF-κB pathway from noncanonical NF-κB signaling in macrophages.Sci Signal. 2016 Dec 6;9(457):ra120. doi: 10.1126/scisignal.aaf1129. Sci Signal. 2016. PMID: 27923915 Free PMC article.
-
Tumor necrosis factor-like weak inducer of apoptosis (TWEAK) promotes glioma cell invasion through induction of NF-κB-inducing kinase (NIK) and noncanonical NF-κB signaling.Mol Cancer. 2015 Jan 27;14(1):9. doi: 10.1186/s12943-014-0273-1. Mol Cancer. 2015. PMID: 25622756 Free PMC article.
-
Noncanonical NF-κB Signaling Pathway in Liver Diseases.J Clin Transl Hepatol. 2021 Feb 28;9(1):81-89. doi: 10.14218/JCTH.2020.00063. Epub 2020 Dec 21. J Clin Transl Hepatol. 2021. PMID: 33604258 Free PMC article. Review.
-
Manipulation of Non-canonical NF-κB Signaling by Non-oncogenic Viruses.Arch Immunol Ther Exp (Warsz). 2019 Feb;67(1):41-48. doi: 10.1007/s00005-018-0522-x. Epub 2018 Sep 8. Arch Immunol Ther Exp (Warsz). 2019. PMID: 30196473 Free PMC article. Review.
Cited by
-
Orthopoxvirus Zoonoses-Do We Still Remember and Are Ready to Fight?Pathogens. 2023 Feb 21;12(3):363. doi: 10.3390/pathogens12030363. Pathogens. 2023. PMID: 36986285 Free PMC article. Review.
References
Grants and funding
LinkOut - more resources
Full Text Sources