MnDPDP: Contrast Agent for Imaging and Protection of Viable Tissue
- PMID: 32994754
- PMCID: PMC7501573
- DOI: 10.1155/2020/3262835
MnDPDP: Contrast Agent for Imaging and Protection of Viable Tissue
Abstract
The semistable chelate manganese (Mn) dipyridoxyl diphosphate (MnDPDP, mangafodipir), previously used as an intravenous (i.v.) contrast agent (Teslascan™, GE Healthcare) for Mn-ion-enhanced MRI (MEMRI), should be reappraised for clinical use but now as a diagnostic drug with cytoprotective properties. Approved for imaging of the liver and pancreas, MnDPDP enhances contrast also in other targets such as the heart, kidney, glandular tissue, and potentially retina and brain. Transmetallation releases paramagnetic Mn2+ for cellular uptake in competition with calcium (Ca2+), and intracellular (IC) macromolecular Mn2+ adducts lower myocardial T 1 to midway between native values and values obtained with gadolinium (Gd3+). What is essential is that T 1 mapping and, to a lesser degree, T 1 weighted imaging enable quantification of viability at a cellular or even molecular level. IC Mn2+ retention for hours provides delayed imaging as another advantage. Examples in humans include quantitative imaging of cardiomyocyte remodeling and of Ca2+ channel activity, capabilities beyond the scope of Gd3+ based or native MRI. In addition, MnDPDP and the metabolite Mn dipyridoxyl diethyl-diamine (MnPLED) act as catalytic antioxidants enabling prevention and treatment of oxidative stress caused by tissue injury and inflammation. Tested applications in humans include protection of normal cells during chemotherapy of cancer and, potentially, of ischemic tissues during reperfusion. Theragnostic use combining therapy with delayed imaging remains to be explored. This review updates MnDPDP and its clinical potential with emphasis on the working mode of an exquisite chelate in the diagnosis of heart disease and in the treatment of oxidative stress.
Copyright © 2020 Per Jynge et al.
Conflict of interest statement
Jynge, Skjold, and Eidsaunet own shares in the Norwegian R&D company IC Targets AS that attempts to reintroduce MnDPDP for diagnostic use. Andersson and Karlsson own shares in the Swedish company PledPharma AB that promotes derivatives of MnDPDP for therapy. Jynge, Skjold, Andersson, and Karlsson are inventors of patents involving MnDPDP for diagnosis and/or therapy. Falkmer, Bruvold, Seland, and Blomlie declare no conflicts of interest.
Figures
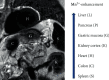
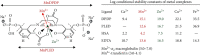
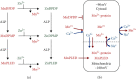
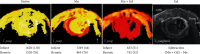
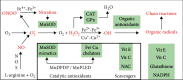
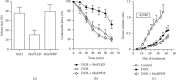
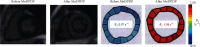
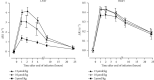
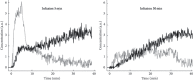
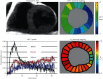
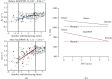
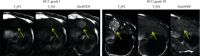
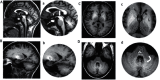
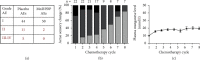
Similar articles
-
Manganese dipyridoxyl diphosphate: MRI contrast agent with antioxidative and cardioprotective properties?Biochem Biophys Res Commun. 1999 Jan 27;254(3):768-72. doi: 10.1006/bbrc.1998.0131. Biochem Biophys Res Commun. 1999. PMID: 9920816
-
Myocardial manganese elevation and proton relaxivity enhancement with manganese dipyridoxyl diphosphate. Ex vivo assessments in normally perfused and ischemic guinea pig hearts.NMR Biomed. 1999 Oct;12(6):364-72. doi: 10.1002/(sici)1099-1492(199910)12:6<364::aid-nbm585>3.0.co;2-z. NMR Biomed. 1999. PMID: 10516618
-
Mangafodipir trisodium injection, a new contrast medium for magnetic resonance imaging: detection and quantitation of the parent compound MnDPDP and metabolites in human plasma by high performance liquid chromatography.J Pharm Biomed Anal. 1997 Apr;15(7):973-81. doi: 10.1016/s0731-7085(96)01945-0. J Pharm Biomed Anal. 1997. PMID: 9160264
-
Calmangafodipir [Ca4Mn(DPDP)5], mangafodipir (MnDPDP) and MnPLED with special reference to their SOD mimetic and therapeutic properties.Drug Discov Today. 2015 Apr;20(4):411-21. doi: 10.1016/j.drudis.2014.11.008. Epub 2014 Nov 20. Drug Discov Today. 2015. PMID: 25463039 Review.
-
Overview of MnDPDP as a pancreas-specific contrast agent for MR imaging.Acta Radiol. 1997 Jul;38(4 Pt 2):660-4. doi: 10.1080/02841859709172398. Acta Radiol. 1997. PMID: 9245961 Review.
Cited by
-
Repeatability and reproducibility of cardiac manganese-enhanced magnetic resonance imaging.Sci Rep. 2023 Feb 27;13(1):3366. doi: 10.1038/s41598-023-29591-z. Sci Rep. 2023. PMID: 36849509 Free PMC article.
-
VHPKQHR Peptide Modified Ultrasmall Paramagnetic Iron Oxide Nanoparticles Targeting Rheumatoid Arthritis for T1-Weighted Magnetic Resonance Imaging.Front Bioeng Biotechnol. 2022 Feb 28;10:821256. doi: 10.3389/fbioe.2022.821256. eCollection 2022. Front Bioeng Biotechnol. 2022. PMID: 35295653 Free PMC article.
-
Manganese-Enhanced Magnetic Resonance Imaging of the Heart.J Magn Reson Imaging. 2023 Apr;57(4):1011-1028. doi: 10.1002/jmri.28499. Epub 2022 Oct 31. J Magn Reson Imaging. 2023. PMID: 36314991 Free PMC article. Review.
References
-
- European Medicines Agency (EMA) PRAC Concludes Assessment of Gadolinium Agents Used in the Body Scans and Recommends Regulatory Action, Including Suspension for Some Marketing Autherizations. Amsterdam, Netherlands: EMA; 2017.
-
- Lauterbur P. C., Mendoca Dias M. H., Rudin A. M. Augmentation of water proton spin-lattice relaxation rates by the in vitro addition of paramagnetic ions. In: Duton P. L., Leigh J. S., Scarpa A., editors. Frontiers of Biological Energetics: Electrons to Tissues. New York, NY, USA: Academic Press; 1978. pp. 752–759. - DOI
Publication types
MeSH terms
Substances
LinkOut - more resources
Full Text Sources
Miscellaneous