Cellular senescence in cancer: from mechanisms to detection
- PMID: 32981205
- PMCID: PMC8486596
- DOI: 10.1002/1878-0261.12807
Cellular senescence in cancer: from mechanisms to detection
Abstract
Senescence refers to a cellular state featuring a stable cell-cycle arrest triggered in response to stress. This response also involves other distinct morphological and intracellular changes including alterations in gene expression and epigenetic modifications, elevated macromolecular damage, metabolism deregulation and a complex pro-inflammatory secretory phenotype. The initial demonstration of oncogene-induced senescence in vitro established senescence as an important tumour-suppressive mechanism, in addition to apoptosis. Senescence not only halts the proliferation of premalignant cells but also facilitates the clearance of affected cells through immunosurveillance. Failure to clear senescent cells owing to deficient immunosurveillance may, however, lead to a state of chronic inflammation that nurtures a pro-tumorigenic microenvironment favouring cancer initiation, migration and metastasis. In addition, senescence is a response to post-therapy genotoxic stress. Therefore, tracking the emergence of senescent cells becomes pivotal to detect potential pro-tumorigenic events. Current protocols for the in vivo detection of senescence require the analysis of fixed or deep-frozen tissues, despite a significant clinical need for real-time bioimaging methods. Accuracy and efficiency of senescence detection are further hampered by a lack of universal and more specific senescence biomarkers. Recently, in an attempt to overcome these hurdles, an assortment of detection tools has been developed. These strategies all have significant potential for clinical utilisation and include flow cytometry combined with histo- or cytochemical approaches, nanoparticle-based targeted delivery of imaging contrast agents, OFF-ON fluorescent senoprobes, positron emission tomography senoprobes and analysis of circulating SASP factors, extracellular vesicles and cell-free nucleic acids isolated from plasma. Here, we highlight the occurrence of senescence in neoplasia and advanced tumours, assess the impact of senescence on tumorigenesis and discuss how the ongoing development of senescence detection tools might improve early detection of multiple cancers and response to therapy in the near future.
Keywords: cancer; cellular senescence; detection; senoprobes; tumour microenvironment.
© 2020 The Authors. Published by FEBS Press and John Wiley & Sons Ltd.
Conflict of interest statement
The authors declare no conflict of interest.
Figures
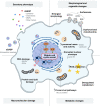
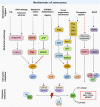
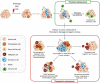
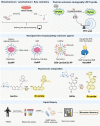
Similar articles
-
The role of cellular senescence and SASP in tumour microenvironment.FEBS J. 2023 Mar;290(5):1348-1361. doi: 10.1111/febs.16381. Epub 2022 Feb 20. FEBS J. 2023. PMID: 35106956 Review.
-
Pro- and anti-tumorigenic functions of the senescence-associated secretory phenotype.Expert Opin Ther Targets. 2019 Dec;23(12):1041-1051. doi: 10.1080/14728222.2019.1565658. Epub 2019 Jan 15. Expert Opin Ther Targets. 2019. PMID: 30616404 Free PMC article. Review.
-
Overcoming the senescence-associated secretory phenotype (SASP): a complex mechanism of resistance in the treatment of cancer.Mol Oncol. 2021 Dec;15(12):3242-3255. doi: 10.1002/1878-0261.13042. Epub 2021 Jul 12. Mol Oncol. 2021. PMID: 34137158 Free PMC article. Review.
-
Exosomes as Emerging Pro-Tumorigenic Mediators of the Senescence-Associated Secretory Phenotype.Int J Mol Sci. 2019 May 24;20(10):2547. doi: 10.3390/ijms20102547. Int J Mol Sci. 2019. PMID: 31137607 Free PMC article. Review.
-
Cellular senescence and the tumour microenvironment.Mol Oncol. 2022 Sep;16(18):3333-3351. doi: 10.1002/1878-0261.13268. Epub 2022 Jun 26. Mol Oncol. 2022. PMID: 35674109 Free PMC article. Review.
Cited by
-
Cellular senescence by loss of Men1 in osteoblasts is critical for age-related osteoporosis.Aging Cell. 2024 Oct;23(10):e14254. doi: 10.1111/acel.14254. Epub 2024 Jun 22. Aging Cell. 2024. PMID: 39384404 Free PMC article.
-
Targeting senescence as an anticancer therapy.Mol Oncol. 2022 Nov;16(21):3855-3880. doi: 10.1002/1878-0261.13312. Epub 2022 Sep 23. Mol Oncol. 2022. PMID: 36065138 Free PMC article. Review.
-
Targeting senescence-associated secretory phenotypes to remodel the tumour microenvironment and modulate tumour outcomes.Clin Transl Med. 2024 Sep;14(9):e1772. doi: 10.1002/ctm2.1772. Clin Transl Med. 2024. PMID: 39270064 Free PMC article. Review.
-
Molecular Analysis of Short- versus Long-Term Survivors of High-Grade Serous Ovarian Carcinoma.Cancers (Basel). 2022 Aug 30;14(17):4198. doi: 10.3390/cancers14174198. Cancers (Basel). 2022. PMID: 36077735 Free PMC article.
-
Cellular senescence in cancer: clinical detection and prognostic implications.J Exp Clin Cancer Res. 2022 Dec 27;41(1):360. doi: 10.1186/s13046-022-02555-3. J Exp Clin Cancer Res. 2022. PMID: 36575462 Free PMC article. Review.
References
-
- Hayflick L & Moorhead PS (1961) The serial cultivation of human diploid cell strains. Exp Cell Res 25, 585–621. - PubMed
-
- Blackburn EH & Gall JG (1978) A tandemly repeated sequence at the termini of the extrachromosomal ribosomal RNA genes in Tetrahymena . J Mol Biol 120, 33–53. - PubMed
-
- Greider CW & Blackburn EH (1985) Identification of a specific telomere terminal transferase activity in tetrahymena extracts. Cell 43, 405–413. - PubMed
-
- Calcinotto A, Kohli J, Zagato E, Pellegrini L, Demaria M & Alimonti A (2019) Cellular senescence: aging, cancer, and injury. Physiol Rev 99, 1047–1078. - PubMed
Publication types
MeSH terms
Substances
Grants and funding
LinkOut - more resources
Full Text Sources
Miscellaneous