Wound Healing Driver Gene and Therapeutic Development: Political and Scientific Hurdles
- PMID: 32966158
- PMCID: PMC8236301
- DOI: 10.1089/wound.2019.1143
Wound Healing Driver Gene and Therapeutic Development: Political and Scientific Hurdles
Abstract
Significance: Since the last Food and Drug Administration (FDA) approval of a wound healing therapeutic in 1997, no new therapeutic candidate (excluding physical therapies, devices, dressings, and antimicrobial agents) has advanced to clinical applications. During this period, the FDA drug approvals for tumors, which have been referred to as "wounds that do not heal," have reached a total of 284 (by end of 2018). Both political and scientific factors may explain this large discrepancy in drug approvals for the two seemingly related and equally complex pathophysiological conditions. Recent Advances: Using the current research funding ratio of 1:150 for wound healing to cancer and the 5% FDA drug approval rate for oncology, we reach a crude estimate of a 0.03% success rate for wound healing therapeutics. Unless a drastic improvement of the current situation, we express a pessimistic outlook toward new and effective wound healing drugs. Critical Issues: We argue that successful development of wound healing therapeutics will rely on identification of wound healing driver genes (WDGs), and the focus should be on WDGs for the wound closure phase of wound healing. Therefore, WDGs must be both necessary and sufficient for wound closure; the absence of a WDG disrupts wound closure, while its supplementation alone is sufficient to restore full wound closure. Successful translation of a WDG into therapeutics requires availability of well-defined animal models with a high degree of relevance to humans. This review discusses the main hurdles faced by the wound healing research community behind the development of so-called "rescuing drugs" for wound healing. Future Directions: Given the lack of new wound healing drugs for the past 23 years, there is a need for a wide range of fresh, innovative, and thorough debates on wound healing drug development, including an organized movement to raise public support for wound healing research.
Keywords: Hsp90; growth factors; necessity and sufficiency; therapeutic; wound closure; wound healing driver gene.
Conflict of interest statement
Authors claim no competing financial interests. All contents of this article were constructed and written by the authors listed. There were absolutely no ghostwriters used for the writing.
Figures
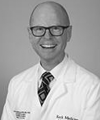
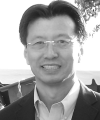
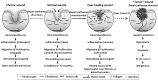
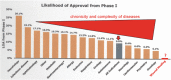
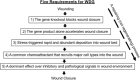
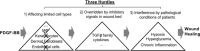
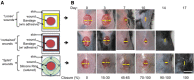
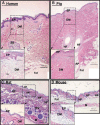
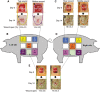
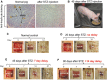
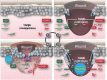
Similar articles
-
Why Are There So Few FDA-Approved Therapeutics for Wound Healing?Int J Mol Sci. 2023 Oct 12;24(20):15109. doi: 10.3390/ijms242015109. Int J Mol Sci. 2023. PMID: 37894789 Free PMC article. Review.
-
Identification of the critical therapeutic entity in secreted Hsp90α that promotes wound healing in newly re-standardized healthy and diabetic pig models.PLoS One. 2014 Dec 2;9(12):e113956. doi: 10.1371/journal.pone.0113956. eCollection 2014. PLoS One. 2014. PMID: 25464502 Free PMC article.
-
The role of secreted heat shock protein-90 (Hsp90) in wound healing - how could it shape future therapeutics?Expert Rev Proteomics. 2017 Aug;14(8):665-675. doi: 10.1080/14789450.2017.1355244. Epub 2017 Jul 31. Expert Rev Proteomics. 2017. PMID: 28715921 Free PMC article. Review.
-
Identification and content validation of wound therapy clinical endpoints relevant to clinical practice and patient values for FDA approval. Part 1. Survey of the wound care community.Wound Repair Regen. 2017 May;25(3):454-465. doi: 10.1111/wrr.12533. Epub 2017 Apr 27. Wound Repair Regen. 2017. PMID: 28370922
-
Management of chronic pressure ulcers: an evidence-based analysis.Ont Health Technol Assess Ser. 2009;9(3):1-203. Epub 2009 Jul 1. Ont Health Technol Assess Ser. 2009. PMID: 23074533 Free PMC article.
Cited by
-
Basic immunologic study as a foundation for engineered therapeutic development.Pharmacol Res Perspect. 2024 Aug;12(4):e1168. doi: 10.1002/prp2.1168. Pharmacol Res Perspect. 2024. PMID: 38894611 Free PMC article. Review.
-
Exploring the impact of TGF-β family gene mutations and expression on skin wound healing and tissue repair.Int Wound J. 2024 Apr;21(4):e14596. doi: 10.1111/iwj.14596. Epub 2023 Dec 27. Int Wound J. 2024. PMID: 38151761 Free PMC article.
-
Discovery of Cell Number-Interstitial Fluid Volume (CIF) Ratio Reveals Secretory Autophagy Pathway to Supply eHsp90α for Wound Healing.Cells. 2024 Jul 30;13(15):1280. doi: 10.3390/cells13151280. Cells. 2024. PMID: 39120311 Free PMC article.
-
Why Are There So Few FDA-Approved Therapeutics for Wound Healing?Int J Mol Sci. 2023 Oct 12;24(20):15109. doi: 10.3390/ijms242015109. Int J Mol Sci. 2023. PMID: 37894789 Free PMC article. Review.
-
Self-assembly of PEG-PPS polymers and LL-37 peptide nanomicelles improves the oxidative microenvironment and promotes angiogenesis to facilitate chronic wound healing.Bioeng Transl Med. 2023 Nov 8;9(2):e10619. doi: 10.1002/btm2.10619. eCollection 2024 Mar. Bioeng Transl Med. 2023. PMID: 38435813 Free PMC article.
References
-
- NCI Director's Update. Annual Report to the National on the Status of Cancer. deainfo.nci.nih.gov/advisory/fac/0619/Lowy.pdf (last accessed May30, 2019)
-
- Center Watch. FDA Approved Drug for Oncology. Copy right 1995–2019. www.centerwatch.com/directories/1067-fda-approved-drugs/topic/103-oncology (last accessed July15, 2020)
-
- Dvorak HF. Tumors: wounds that do not heal. N Engl J Med 1986;315:1650–1659 - PubMed
-
- Michelotti GA, Machado MV, Diehl AM. NAFLD, NASH and liver cancer. Nat Rev Gastroenterol Hepatol 2013;10:656. - PubMed
-
- Diehl AM, Day C. Cause, pathogenesis, and treatment of nonalcoholic steatohepatitis. N Engl J Med 2017;377:2063–2072 - PubMed
Publication types
MeSH terms
Substances
Grants and funding
LinkOut - more resources
Full Text Sources
Medical
Miscellaneous