Regulation of lymphatic function and injury by nitrosative stress in obese mice
- PMID: 32941994
- PMCID: PMC7536739
- DOI: 10.1016/j.molmet.2020.101081
Regulation of lymphatic function and injury by nitrosative stress in obese mice
Abstract
Objective: Obesity results in lymphatic dysfunction, but the cellular mechanisms that mediate this effect remain largely unknown. Previous studies in obese mice have shown that inducible nitric oxide synthase-expressing (iNOS+) inflammatory cells accumulate around lymphatic vessels. In the current study, we therefore tested the hypothesis that increased expression of iNOS results in nitrosative stress and injury to the lymphatic endothelial cells (LECs). In addition, we tested the hypothesis that lymphatic injury, independent of obesity, can modulate glucose and lipid metabolism.
Methods: We compared the metabolic changes and lymphatic function of wild-type and iNOS knockout mice fed a normal chow or high-fat diet for 16 weeks. To corroborate our in vivo findings, we analyzed the effects of reactive nitrogen species on isolated LECs. Finally, using a genetically engineered mouse model that allows partial ablation of the lymphatic system, we studied the effects of acute lymphatic injury on glucose and lipid metabolism in lean mice.
Results: The mesenteric lymphatic vessels of obese wild-type animals were dilated, leaky, and surrounded by iNOS+ inflammatory cells with resulting increased accumulation of reactive nitrogen species when compared with lean wild-type or obese iNOS knockout animals. These changes in obese wild-type mice were associated with systemic glucose and lipid abnormalities, as well as decreased mesenteric LEC expression of lymphatic-specific genes, including vascular endothelial growth factor receptor 3 (VEGFR-3) and antioxidant genes as compared with lean wild-type or obese iNOS knockout animals. In vitro experiments demonstrated that isolated LECs were more sensitive to reactive nitrogen species than blood endothelial cells, and that this sensitivity was ameliorated by antioxidant therapies. Finally, using mice in which the lymphatics were specifically ablated using diphtheria toxin, we found that the interaction between metabolic abnormalities caused by obesity and lymphatic dysfunction is bidirectional. Targeted partial ablation of mesenteric lymphatic channels of lean mice resulted in increased accumulation of iNOS+ inflammatory cells and increased reactive nitrogen species. Lymphatic ablation also caused marked abnormalities in insulin sensitivity, serum glucose and insulin concentrations, expression of insulin-sensitive genes, lipid metabolism, and significantly increased systemic and mesenteric white adipose tissue (M-WAT) inflammatory responses.
Conclusions: Our studies suggest that increased iNOS production in obese animals plays a key role in regulating lymphatic injury by increasing nitrosative stress. In addition, our studies suggest that obesity-induced lymphatic injury may amplify metabolic abnormalities by increasing systemic and local inflammatory responses and regulating insulin sensitivity. These findings suggest that manipulation of the lymphatic system may represent a novel means of treating metabolic abnormalities associated with obesity.
Keywords: Inflammation; Lymphatic function; Metabolism; Nitrosative stress; Obesity; iNOS.
Copyright © 2020 The Authors. Published by Elsevier GmbH.. All rights reserved.
Figures
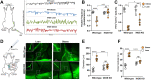
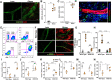
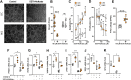
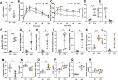
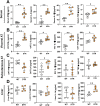
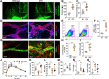
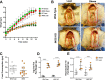
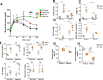
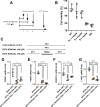
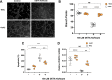
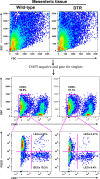
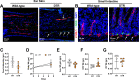
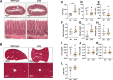
Similar articles
-
Obese mice lacking inducible nitric oxide synthase are sensitized to the metabolic actions of peroxisome proliferator-activated receptor-gamma agonism.Diabetes. 2008 Aug;57(8):1999-2011. doi: 10.2337/db08-0540. Epub 2008 May 5. Diabetes. 2008. PMID: 18458147 Free PMC article.
-
Lymphatic endothelial cells efferent to inflamed joints produce iNOS and inhibit lymphatic vessel contraction and drainage in TNF-induced arthritis in mice.Arthritis Res Ther. 2016 Mar 12;18:62. doi: 10.1186/s13075-016-0963-8. Arthritis Res Ther. 2016. PMID: 26970913 Free PMC article.
-
Hepatic Lysosomal iNOS Activity Impairs Autophagy in Obesity.Cell Mol Gastroenterol Hepatol. 2019;8(1):95-110. doi: 10.1016/j.jcmgh.2019.03.005. Epub 2019 Mar 27. Cell Mol Gastroenterol Hepatol. 2019. PMID: 30926581 Free PMC article.
-
The Lymphatic Vasculature: Its Role in Adipose Metabolism and Obesity.Cell Metab. 2017 Oct 3;26(4):598-609. doi: 10.1016/j.cmet.2017.07.020. Epub 2017 Aug 24. Cell Metab. 2017. PMID: 28844882 Free PMC article. Review.
-
Effects of diet-induced obesity in the development of lymphedema in the animal model: A literature review.Obes Res Clin Pract. 2022 May-Jun;16(3):197-205. doi: 10.1016/j.orcp.2022.05.003. Epub 2022 Jun 2. Obes Res Clin Pract. 2022. PMID: 35659463 Review.
Cited by
-
Reactive Oxygen Species in Regulating Lymphangiogenesis and Lymphatic Function.Cells. 2022 May 26;11(11):1750. doi: 10.3390/cells11111750. Cells. 2022. PMID: 35681445 Free PMC article. Review.
-
Significant Decrease in Glycated Hemoglobin, 2h-Post-Load Glucose and High-Sensitivity C-Reactive Protein Levels in Patients with Abnormal Body Mass Index after Therapy with Manual Lymphatic Drainage.Biomedicines. 2022 Jul 18;10(7):1730. doi: 10.3390/biomedicines10071730. Biomedicines. 2022. PMID: 35885034 Free PMC article.
-
Junctional integrity and directional mobility of lymphatic endothelial cell monolayers are disrupted by saturated fatty acids.Mol Biol Cell. 2023 Apr 1;34(4):ar28. doi: 10.1091/mbc.E22-08-0367. Epub 2023 Feb 3. Mol Biol Cell. 2023. PMID: 36735487 Free PMC article.
-
Edema and lymphatic clearance: molecular mechanisms and ongoing challenges.Clin Sci (Lond). 2023 Sep 27;137(18):1451-1476. doi: 10.1042/CS20220314. Clin Sci (Lond). 2023. PMID: 37732545 Free PMC article. Review.
-
Lymphatic Vascular Permeability.Cold Spring Harb Perspect Med. 2022 Aug 15;12(8):a041274. doi: 10.1101/cshperspect.a041274. Cold Spring Harb Perspect Med. 2022. PMID: 35879102 Free PMC article. Review.
References
-
- Harvey N.L., Srinivasan R.S., Dillard M.E., Johnson N.C., Witte M.H., Boyd K. Lymphatic vascular defects promoted by Prox1 haploinsufficiency cause adult-onset obesity. Nature Genetics. 2005;37(10):1072–1081. - PubMed
Publication types
MeSH terms
Substances
Grants and funding
LinkOut - more resources
Full Text Sources
Molecular Biology Databases
Miscellaneous