Xenotropic and polytropic retrovirus receptor 1 regulates procoagulant platelet polyphosphate
- PMID: 32932519
- PMCID: PMC7955403
- DOI: 10.1182/blood.2019004617
Xenotropic and polytropic retrovirus receptor 1 regulates procoagulant platelet polyphosphate
Abstract
Polyphosphate is a procoagulant inorganic polymer of linear-linked orthophosphate residues. Multiple investigations have established the importance of platelet polyphosphate in blood coagulation; however, the mechanistic details of polyphosphate homeostasis in mammalian species remain largely undefined. In this study, xenotropic and polytropic retrovirus receptor 1 (XPR1) regulated polyphosphate in platelets and was implicated in thrombosis in vivo. We used bioinformatic analyses of omics data to identify XPR1 as a major phosphate transporter in platelets. XPR1 messenger RNA and protein expression inversely correlated with intracellular polyphosphate content and release. Pharmacological interference with XPR1 activity increased polyphosphate stores, led to enhanced platelet-driven coagulation, and amplified thrombus formation under flow via the polyphosphate/factor XII pathway. Conditional gene deletion of Xpr1 in platelets resulted in polyphosphate accumulation, accelerated arterial thrombosis, and augmented activated platelet-driven pulmonary embolism without increasing bleeding in mice. These data identify platelet XPR1 as an integral regulator of platelet polyphosphate metabolism and reveal a fundamental role for phosphate homeostasis in thrombosis.
Conflict of interest statement
Conflict-of-interest disclosure: The authors declare no competing financial interests.
Figures
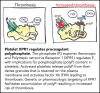
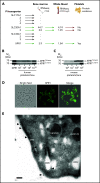
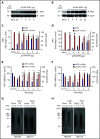
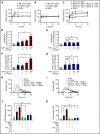
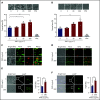
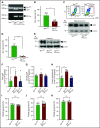
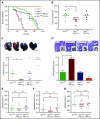
Comment in
-
XPoRting (poly)phosphates limits thrombosis.Blood. 2021 Mar 11;137(10):1278-1280. doi: 10.1182/blood.2020008973. Blood. 2021. PMID: 33704397 No abstract available.
Similar articles
-
Platelet polyphosphates: the nexus of primary and secondary hemostasis.Scand J Clin Lab Invest. 2011 Apr;71(2):82-6. doi: 10.3109/00365513.2010.550312. Scand J Clin Lab Invest. 2011. PMID: 21385010 Review.
-
Polyphosphate nanoparticles on the platelet surface trigger contact system activation.Blood. 2017 Mar 23;129(12):1707-1717. doi: 10.1182/blood-2016-08-734988. Epub 2017 Jan 3. Blood. 2017. PMID: 28049643 Free PMC article.
-
Platelet polyphosphates are proinflammatory and procoagulant mediators in vivo.Cell. 2009 Dec 11;139(6):1143-56. doi: 10.1016/j.cell.2009.11.001. Cell. 2009. PMID: 20005807 Free PMC article.
-
Interplay between primary familial brain calcification-associated SLC20A2 and XPR1 phosphate transporters requires inositol polyphosphates for control of cellular phosphate homeostasis.J Biol Chem. 2020 Jul 10;295(28):9366-9378. doi: 10.1074/jbc.RA119.011376. Epub 2020 May 11. J Biol Chem. 2020. PMID: 32393577 Free PMC article.
-
The polyphosphate/factor XII pathway in cancer-associated thrombosis: novel perspectives for safe anticoagulation in patients with malignancies.Thromb Res. 2016 May;141 Suppl 2:S4-7. doi: 10.1016/S0049-3848(16)30353-X. Thromb Res. 2016. PMID: 27207422 Review.
Cited by
-
The contact system in liver injury.Semin Immunopathol. 2021 Aug;43(4):507-517. doi: 10.1007/s00281-021-00876-7. Epub 2021 Jun 14. Semin Immunopathol. 2021. PMID: 34125270 Free PMC article. Review.
-
Homeostatic coordination of cellular phosphate uptake and efflux requires an organelle-based receptor for the inositol pyrophosphate IP8.Cell Rep. 2024 Jun 25;43(6):114316. doi: 10.1016/j.celrep.2024.114316. Epub 2024 Jun 2. Cell Rep. 2024. PMID: 38833370 Free PMC article.
-
Identification of the factor XII contact activation site enables sensitive coagulation diagnostics.Nat Commun. 2021 Sep 22;12(1):5596. doi: 10.1038/s41467-021-25888-7. Nat Commun. 2021. PMID: 34552086 Free PMC article.
-
Plant phosphate nutrition: sensing the stress.Stress Biol. 2022 Mar 3;2(1):16. doi: 10.1007/s44154-022-00039-0. Stress Biol. 2022. PMID: 37676547 Free PMC article. Review.
-
Epigenetic modification: A novel insight into diabetic wound healing.Heliyon. 2024 Mar 13;10(6):e28086. doi: 10.1016/j.heliyon.2024.e28086. eCollection 2024 Mar 30. Heliyon. 2024. PMID: 38533007 Free PMC article. Review.
References
-
- Rao NN, Gómez-García MR, Kornberg A. Inorganic polyphosphate: essential for growth and survival. Annu Rev Biochem. 2009;78(1):605-647. - PubMed
-
- Igor S, Kulaev VV. The Biochemistry of Inorganic Polyphosphates. 2nd ed. Chichester: John Wiley & Sons; 2004.
-
- Secco D, Wang C, Shou H, Whelan J. Phosphate homeostasis in the yeast Saccharomyces cerevisiae, the key role of the SPX domain-containing proteins. FEBS Lett. 2012;586(4):289-295. - PubMed
Publication types
MeSH terms
Substances
Grants and funding
LinkOut - more resources
Full Text Sources
Other Literature Sources
Medical
Molecular Biology Databases