Cell Mechanics at the Rear Act to Steer the Direction of Cell Migration
- PMID: 32916096
- PMCID: PMC7530145
- DOI: 10.1016/j.cels.2020.08.008
Cell Mechanics at the Rear Act to Steer the Direction of Cell Migration
Abstract
Motile cells navigate complex environments by changing their direction of travel, generating left-right asymmetries in their mechanical subsystems to physically turn. Currently, little is known about how external directional cues are propagated along the length scale of the whole cell and integrated with its force-generating apparatus to steer migration mechanically. We examine the mechanics of spontaneous cell turning in fish epidermal keratocytes and find that the mechanical asymmetries responsible for turning behavior predominate at the rear of the cell, where there is asymmetric centripetal actin flow. Using experimental perturbations, we identify two linked feedback loops connecting myosin II contractility, adhesion strength and actin network flow in turning cells that are sufficient to explain the observed cell shapes and trajectories. Notably, asymmetries in actin polymerization at the cell leading edge play only a minor role in the mechanics of cell turning-that is, cells steer from the rear.
Keywords: actin; adhesion; asymmetry; cell migration; cell motility; cell turning; keratocyte; myosin; self-organization.
Copyright © 2020 Elsevier Inc. All rights reserved.
Conflict of interest statement
Declaration of Interests The authors declare no competing interests.
Figures
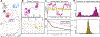
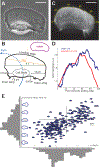
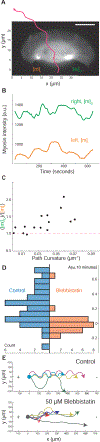
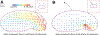
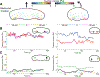
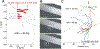
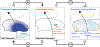
Similar articles
-
A free-boundary model of a motile cell explains turning behavior.PLoS Comput Biol. 2017 Nov 14;13(11):e1005862. doi: 10.1371/journal.pcbi.1005862. eCollection 2017 Nov. PLoS Comput Biol. 2017. PMID: 29136638 Free PMC article.
-
Rear actomyosin contractility-driven directional cell migration in three-dimensional matrices: a mechano-chemical coupling mechanism.J R Soc Interface. 2014 Mar 19;11(95):20131072. doi: 10.1098/rsif.2013.1072. Print 2014 Jun 6. J R Soc Interface. 2014. PMID: 24647903 Free PMC article. Review.
-
Traction force microscopy in rapidly moving cells reveals separate roles for ROCK and MLCK in the mechanics of retraction.Exp Cell Res. 2014 Aug 15;326(2):280-94. doi: 10.1016/j.yexcr.2014.04.015. Epub 2014 Apr 29. Exp Cell Res. 2014. PMID: 24786318
-
Emergence of large-scale cell morphology and movement from local actin filament growth dynamics.PLoS Biol. 2007 Sep;5(9):e233. doi: 10.1371/journal.pbio.0050233. PLoS Biol. 2007. PMID: 17760506 Free PMC article.
-
Experiment, theory, and the keratocyte: An ode to a simple model for cell motility.Semin Cell Dev Biol. 2020 Apr;100:143-151. doi: 10.1016/j.semcdb.2019.10.019. Epub 2019 Nov 9. Semin Cell Dev Biol. 2020. PMID: 31718950 Review.
Cited by
-
Crawling, waving, inch worming, dilating, and pivoting mechanics of migrating cells: Lessons from Ken Jacobson.Biophys J. 2023 Sep 19;122(18):3551-3559. doi: 10.1016/j.bpj.2023.03.023. Epub 2023 Mar 17. Biophys J. 2023. PMID: 36934300 Free PMC article. Review.
-
Coupling cell shape and velocity leads to oscillation and circling in keratocyte galvanotaxis.Biophys J. 2023 Jan 3;122(1):130-142. doi: 10.1016/j.bpj.2022.11.021. Epub 2022 Nov 17. Biophys J. 2023. PMID: 36397670 Free PMC article.
-
Sensing the shape of a cell with reaction diffusion and energy minimization.Proc Natl Acad Sci U S A. 2022 Aug 2;119(31):e2121302119. doi: 10.1073/pnas.2121302119. Epub 2022 Jul 29. Proc Natl Acad Sci U S A. 2022. PMID: 35905323 Free PMC article.
-
Keratocytes migrate against flow with a roly-poly-like mechanism.Proc Natl Acad Sci U S A. 2022 Nov 29;119(48):e2210379119. doi: 10.1073/pnas.2210379119. Epub 2022 Nov 21. Proc Natl Acad Sci U S A. 2022. PMID: 36409912 Free PMC article.
-
Deterministic actin waves as generators of cell polarization cues.Proc Natl Acad Sci U S A. 2020 Jan 14;117(2):826-835. doi: 10.1073/pnas.1907845117. Epub 2019 Dec 27. Proc Natl Acad Sci U S A. 2020. PMID: 31882452 Free PMC article.
References
Publication types
MeSH terms
Grants and funding
LinkOut - more resources
Full Text Sources