Progesterone receptor membrane associated component 1 enhances obesity progression in mice by facilitating lipid accumulation in adipocytes
- PMID: 32887925
- PMCID: PMC7473863
- DOI: 10.1038/s42003-020-01202-x
Progesterone receptor membrane associated component 1 enhances obesity progression in mice by facilitating lipid accumulation in adipocytes
Abstract
Progesterone receptor membrane associated component 1 (PGRMC1) exhibits haem-dependent dimerization on cell membrane and binds to EGF receptor and cytochromes P450 to regulate cancer proliferation and chemoresistance. However, its physiological functions remain unknown. Herein, we demonstrate that PGRMC1 is required for adipogenesis, and its expression is significantly enhanced by insulin or thiazolidine, an agonist for PPARγ. The haem-dimerized PGRMC1 interacts with low-density lipoprotein receptors (VLDL-R and LDL-R) or GLUT4 to regulate their translocation to the plasma membrane, facilitating lipid uptake and accumulation, and de-novo fatty acid synthesis in adipocytes. These events are cancelled by CO through interfering with PGRMC1 dimerization. PGRMC1 expression in mouse adipose tissues is enhanced during obesity induced by a high fat diet. Furthermore, adipose tissue-specific PGRMC1 knockout in mice dramatically suppressed high-fat-diet induced adipocyte hypertrophy. Our results indicate a pivotal role of PGRMC1 in developing obesity through its metabolic regulation of lipids and carbohydrates in adipocytes.
Conflict of interest statement
The authors declare no competing interests.
Figures
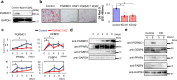
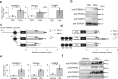
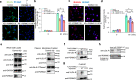
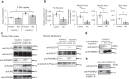
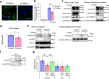
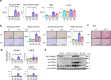
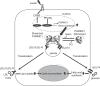
Similar articles
-
LMO3 reprograms visceral adipocyte metabolism during obesity.J Mol Med (Berl). 2021 Aug;99(8):1151-1171. doi: 10.1007/s00109-021-02089-9. Epub 2021 May 20. J Mol Med (Berl). 2021. PMID: 34018016 Free PMC article.
-
Suppression of adipocyte differentiation and lipid accumulation by stearidonic acid (SDA) in 3T3-L1 cells.Lipids Health Dis. 2017 Sep 25;16(1):181. doi: 10.1186/s12944-017-0574-7. Lipids Health Dis. 2017. PMID: 28946872 Free PMC article.
-
Coprinus comatus cap inhibits adipocyte differentiation via regulation of PPARγ and Akt signaling pathway.PLoS One. 2014 Sep 2;9(9):e105809. doi: 10.1371/journal.pone.0105809. eCollection 2014. PLoS One. 2014. PMID: 25181477 Free PMC article.
-
Cystathionine β-synthase and PGRMC1 as CO sensors.Free Radic Biol Med. 2016 Oct;99:333-344. doi: 10.1016/j.freeradbiomed.2016.08.025. Epub 2016 Aug 24. Free Radic Biol Med. 2016. PMID: 27565814 Review.
-
PGRMC1: An enigmatic heme-binding protein.Pharmacol Ther. 2023 Jan;241:108326. doi: 10.1016/j.pharmthera.2022.108326. Epub 2022 Dec 1. Pharmacol Ther. 2023. PMID: 36463977 Free PMC article. Review.
Cited by
-
PPARγ-Induced Global H3K27 Acetylation Maintains Osteo/Cementogenic Abilities of Periodontal Ligament Fibroblasts.Int J Mol Sci. 2021 Aug 11;22(16):8646. doi: 10.3390/ijms22168646. Int J Mol Sci. 2021. PMID: 34445348 Free PMC article.
-
Cross-Linking Mass Spectrometry Uncovers Interactions Between High-Density Lipoproteins and the SARS-CoV-2 Spike Glycoprotein.Mol Cell Proteomics. 2023 Aug;22(8):100600. doi: 10.1016/j.mcpro.2023.100600. Epub 2023 Jun 19. Mol Cell Proteomics. 2023. PMID: 37343697 Free PMC article.
-
Pleiotropy of Progesterone Receptor Membrane Component 1 in Modulation of Cytochrome P450 Activity.J Xenobiot. 2024 May 1;14(2):575-603. doi: 10.3390/jox14020034. J Xenobiot. 2024. PMID: 38804287 Free PMC article. Review.
-
Beyond reproduction: unraveling the impact of sex hormones on cardiometabolic health.Med Rev (2021). 2024 May 23;4(4):284-300. doi: 10.1515/mr-2024-0012. eCollection 2024 Aug. Med Rev (2021). 2024. PMID: 39135604 Free PMC article. Review.
-
Clozapine Induced Disturbances in Hepatic Glucose Metabolism: The Potential Role of PGRMC1 Signaling.Front Endocrinol (Lausanne). 2021 Dec 14;12:727371. doi: 10.3389/fendo.2021.727371. eCollection 2021. Front Endocrinol (Lausanne). 2021. PMID: 34970218 Free PMC article.
References
-
- Lowell BB, Spiegelman BM. Towards a molecular understanding of adaptive thermogenesis. Nature. 2000;404:652–660. - PubMed
-
- Ferreira AV, Menezes-Garcia Z, Viana JB, Mario EG, Botion LM. Distinct metabolic pathways trigger adipocyte fat accumulation induced by high-carbohydrate and high-fat diets. Nutrition. 2014;30:1138–1143. - PubMed
-
- Lefterova MI, Lazar MA. New developments in adipogenesis. Trends Endocrinol. Metab. 2009;20:107–114. - PubMed
-
- Ali AT, Hochfeld WE, Myburgh R, Pepper MS. Adipocyte and adipogenesis. Eur. J. Cell Biol. 2013;92:229–236. - PubMed
-
- Tontonoz P, Spiegelman BM. Fat and beyond: the diverse biology of PPARgamma. Annu. Rev. Biochem. 2008;77:289–312. - PubMed
Publication types
MeSH terms
Substances
LinkOut - more resources
Full Text Sources
Other Literature Sources
Medical
Molecular Biology Databases
Research Materials