Biomechanics of In-Stance Balancing Responses Following Outward-Directed Perturbation to the Pelvis During Very Slow Treadmill Walking Show Complex and Well-Orchestrated Reaction of Central Nervous System
- PMID: 32850738
- PMCID: PMC7399078
- DOI: 10.3389/fbioe.2020.00884
Biomechanics of In-Stance Balancing Responses Following Outward-Directed Perturbation to the Pelvis During Very Slow Treadmill Walking Show Complex and Well-Orchestrated Reaction of Central Nervous System
Abstract
Multiple strategies may be used when counteracting loss of balance during walking. Placing the foot onto a new location is not efficient when walking speed is very low. Instead medio-lateral displacement of center-of-pressure, rotation of body segments to produce a lateral ground-reaction-force, and pronounced braking of movement in the plane of progression is used. It is, however, presently not known in what way these in-stance balancing strategies are interrelated. Twelve healthy subjects walked very slowly on an instrumented treadmill and received outward-directed pushes to the waist. We created experimental conditions where the use of stepping strategy to recover balance following an outward push was minimized by appropriately selecting the amplitude and timing of perturbation. Our experimental results showed that in the first part of the response the principal strategy used to counteract the effect of a perturbing push was a short but substantial increase in lateral ground-reaction-force. Concomitant slowing of the movement and related anterior displacement of center-of-pressure enabled lateral displacement of center-of-pressure which was, together with a short but substantial increase in vertical ground-reaction-force, instrumental in reducing the inevitable increase of whole-body angular momentum in the frontal plane. However, anterior displacement of center-of-pressure and increased vertical ground-reaction-force also induced an increase in whole-body angular momentum in the sagittal plane. In the second part of the response the lateral ground-reaction-force was decreased with respect to unperturbed walking thus allowing for a decrease of whole-body angular momentum in the frontal plane. Additionally, an increase in anterior ground-reaction-force in the second part of the response propelled the center-of-mass in the direction of movement, thus re-synchronizing it with the frontal plane component of the center-of-mass as well as decreasing whole-body angular momentum in the sagittal plane. The results of this study show that use of in-stance balancing strategies counteracts the effect a perturbing push imposed on the center-of-mass, re-synchronizes the movement of center-of-mass in sagittal and frontal planes to the values seen in unperturbed walking and maintains control of whole-body angular momentum in both frontal and sagittal planes.
Keywords: braking strategy; inertial strategy; medio-lateral ankle strategy; perturbed walking; stepping strategy.
Copyright © 2020 Matjačić, Zadravec and Olenšek.
Figures
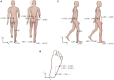
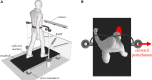
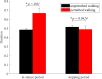
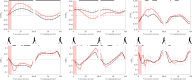
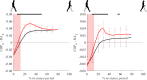
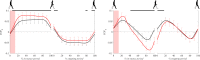
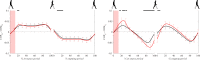
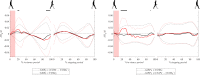
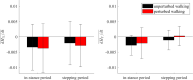
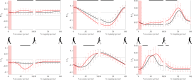
Similar articles
-
Dynamic balancing responses in unilateral transtibial amputees following outward-directed perturbations during slow treadmill walking differ considerably for amputated and non-amputated side.J Neuroeng Rehabil. 2021 Jul 31;18(1):123. doi: 10.1186/s12984-021-00914-3. J Neuroeng Rehabil. 2021. PMID: 34332595 Free PMC article.
-
Influence of Treadmill Speed and Perturbation Intensity on Selection of Balancing Strategies during Slow Walking Perturbed in the Frontal Plane.Appl Bionics Biomech. 2019 Jun 2;2019:1046459. doi: 10.1155/2019/1046459. eCollection 2019. Appl Bionics Biomech. 2019. PMID: 31281413 Free PMC article.
-
An effective balancing response to lateral perturbations at pelvis level during slow walking requires control in all three planes of motion.J Biomech. 2017 Jul 26;60:79-90. doi: 10.1016/j.jbiomech.2017.06.020. Epub 2017 Jun 21. J Biomech. 2017. PMID: 28669548
-
Contributions to the understanding of gait control.Dan Med J. 2014 Apr;61(4):B4823. Dan Med J. 2014. PMID: 24814597 Review.
-
Foot strike alters ground reaction force and knee load when stepping down during ongoing walking.Gait Posture. 2020 Feb;76:327-333. doi: 10.1016/j.gaitpost.2019.12.019. Epub 2019 Dec 24. Gait Posture. 2020. PMID: 31896535 Review.
Cited by
-
Cross-step detection using center-of-pressure based algorithm for real-time applications.J Neuroeng Rehabil. 2024 Sep 16;21(1):161. doi: 10.1186/s12984-024-01460-4. J Neuroeng Rehabil. 2024. PMID: 39285381 Free PMC article.
-
Lower extremity joint compensatory effects during the first recovery step following slipping and stumbling perturbations in young and older subjects.BMC Geriatr. 2022 Aug 10;22(1):656. doi: 10.1186/s12877-022-03354-3. BMC Geriatr. 2022. PMID: 35948887 Free PMC article.
-
Dynamic balancing responses in unilateral transtibial amputees following outward-directed perturbations during slow treadmill walking differ considerably for amputated and non-amputated side.J Neuroeng Rehabil. 2021 Jul 31;18(1):123. doi: 10.1186/s12984-021-00914-3. J Neuroeng Rehabil. 2021. PMID: 34332595 Free PMC article.
References
-
- Bonnefoy-Mazure A., Armand S. (2015). “Normal gait,” in Orthopedic Management of Children with Cerebral Palsy, eds Canavese F., Deslandes J. (Hauppauge, NY: Nova Science Publishers; ), 199–213.
LinkOut - more resources
Full Text Sources