The Heat Shock Protein 27 Immune Complex Enhances Exosomal Cholesterol Efflux
- PMID: 32824555
- PMCID: PMC7460488
- DOI: 10.3390/biomedicines8080290
The Heat Shock Protein 27 Immune Complex Enhances Exosomal Cholesterol Efflux
Abstract
Previously, we demonstrated that Heat Shock Protein 27 (HSP27) reduces the inflammatory stages of experimental atherogenesis, is released by macrophage (MΦ) exosomes and lowers cholesterol levels in atherosclerotic plaques. Recently, we discovered that natural autoantibodies directed against HSP27 enhance its signaling effects, as HSP27 immune complexes (IC) interact at the cell membrane to modulate signaling. We now seek to evaluate the potential role of the HSP27 IC on MΦ exosomal release and cholesterol export. First, in human blood samples, we show that healthy control subjects have 86% more exosomes compared to patients with coronary artery disease (p < 0.0001). Treating human THP-1 MΦ with rHSP27 plus a validated anti-HPS27 IgG antibody increased the abundance of exosomes in the culture media (+98%; p < 0.0001) as well as expression of Flotillin-2, a marker reflective of exosomal release. Exosome cholesterol efflux was independent of Apo-A1. THP-1 MΦ loaded with NBD-labeled cholesterol and treated with the HSP27 IC showed a 22% increase in extracellular vesicles labeled with NBD and a 95% increase in mean fluorescent intensity. In conclusion, exosomal abundance and secretion of cholesterol content increases in response to HSP27 IC treatment, which may represent an important therapeutic option for diseases characterized by cholesterol accumulation.
Keywords: Heat Shock Protein 27; antibody; exosome; immune complex.
Conflict of interest statement
EOB is the Scientific Cofounder of Pemi31 Therapeutics Inc., a start-up company that is developing HSP27 immunotherapeutics. EOB and CS have equity interests in Pemi31 Therapeutics Inc.
Figures
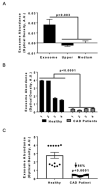
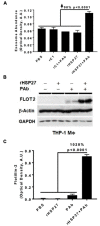
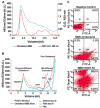
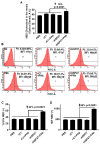
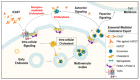
Similar articles
-
Heat shock protein 27 immune complex altered signaling and transport (ICAST): Novel mechanisms of attenuating inflammation.FASEB J. 2020 Nov;34(11):14287-14301. doi: 10.1096/fj.202001389RR. Epub 2020 Sep 4. FASEB J. 2020. PMID: 32888229
-
Characterization of heat shock protein 27 in extracellular vesicles: a potential anti-inflammatory therapy.FASEB J. 2019 Feb;33(2):1617-1630. doi: 10.1096/fj.201800987R. Epub 2018 Sep 6. FASEB J. 2019. PMID: 30188755
-
Unlike estrogens that increase PCSK9 levels post-menopause HSP27 vaccination lowers cholesterol levels and atherogenesis due to divergent effects on PCSK9 and LDLR.Pharmacol Res. 2020 Nov;161:105222. doi: 10.1016/j.phrs.2020.105222. Epub 2020 Oct 3. Pharmacol Res. 2020. PMID: 33022407
-
Extracellular Release and Signaling by Heat Shock Protein 27: Role in Modifying Vascular Inflammation.Front Immunol. 2016 Jul 26;7:285. doi: 10.3389/fimmu.2016.00285. eCollection 2016. Front Immunol. 2016. PMID: 27507972 Free PMC article. Review.
-
Extracellular small heat shock proteins: exosomal biogenesis and function.Cell Stress Chaperones. 2018 May;23(3):441-454. doi: 10.1007/s12192-017-0856-z. Epub 2017 Oct 30. Cell Stress Chaperones. 2018. PMID: 29086335 Free PMC article. Review.
Cited by
-
Mechanistic insights into heat shock protein 27, a potential therapeutic target for cardiovascular diseases.Front Cardiovasc Med. 2023 May 12;10:1195464. doi: 10.3389/fcvm.2023.1195464. eCollection 2023. Front Cardiovasc Med. 2023. PMID: 37252119 Free PMC article. Review.
-
[M2 macrophage-derived exosomal lncRNA NR_028113.1 promotes macrophage polarization possibly by activating the JAK2/STAT3 signaling pathway].Nan Fang Yi Ke Da Xue Xue Bao. 2023 Mar 20;43(3):393-399. doi: 10.12122/j.issn.1673-4254.2023.03.08. Nan Fang Yi Ke Da Xue Xue Bao. 2023. PMID: 37087583 Free PMC article. Chinese.
-
Exosomes in Cardiovascular Disease: From Mechanism to Therapeutic Target.Metabolites. 2023 Mar 27;13(4):479. doi: 10.3390/metabo13040479. Metabolites. 2023. PMID: 37110138 Free PMC article. Review.
-
Enhancing the Cellular Production of Extracellular Vesicles for Developing Therapeutic Applications.Pharm Res. 2023 Apr;40(4):833-853. doi: 10.1007/s11095-022-03420-w. Epub 2022 Nov 1. Pharm Res. 2023. PMID: 36319886 Free PMC article. Review.
-
Basic Pathogenic Mechanisms and Epigenetic Players Promoted by Extracellular Vesicles in Vascular Damage.Int J Mol Sci. 2023 Apr 19;24(8):7509. doi: 10.3390/ijms24087509. Int J Mol Sci. 2023. PMID: 37108672 Free PMC article. Review.
References
LinkOut - more resources
Full Text Sources
Research Materials
Miscellaneous