Biomedical Applications of Tissue Clearing and Three-Dimensional Imaging in Health and Disease
- PMID: 32805648
- PMCID: PMC7452225
- DOI: 10.1016/j.isci.2020.101432
Biomedical Applications of Tissue Clearing and Three-Dimensional Imaging in Health and Disease
Abstract
Three-dimensional (3D) optical imaging techniques can expand our knowledge about physiological and pathological processes that cannot be fully understood with 2D approaches. Standard diagnostic tests frequently are not sufficient to unequivocally determine the presence of a pathological condition. Whole-organ optical imaging requires tissue transparency, which can be achieved by using tissue clearing procedures enabling deeper image acquisition and therefore making possible the analysis of large-scale biological tissue samples. Here, we review currently available clearing agents, methods, and their application in imaging of physiological or pathological conditions in different animal and human organs. We also compare different optical tissue clearing methods discussing their advantages and disadvantages and review the use of different 3D imaging techniques for the visualization and image acquisition of cleared tissues. The use of optical tissue clearing resources for large-scale biological tissues 3D imaging paves the way for future applications in translational and clinical research.
Keywords: Biomedical Discipline; Imaging Methods in Chemistry; Medical Imaging; Optical Imaging.
Copyright © 2020 The Authors. Published by Elsevier Inc. All rights reserved.
Figures
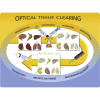
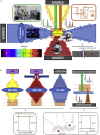
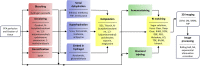
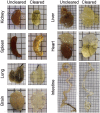
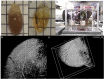
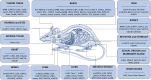
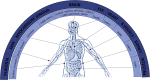
Similar articles
-
Considerations for using optical clearing techniques for 3D imaging of nanoparticle biodistribution.Int J Pharm. 2020 Oct 15;588:119739. doi: 10.1016/j.ijpharm.2020.119739. Epub 2020 Aug 9. Int J Pharm. 2020. PMID: 32783979 Review.
-
Imaging the mammary gland and mammary tumours in 3D: optical tissue clearing and immunofluorescence methods.Breast Cancer Res. 2016 Dec 13;18(1):127. doi: 10.1186/s13058-016-0754-9. Breast Cancer Res. 2016. PMID: 27964754 Free PMC article.
-
Tissue optical clearing for 3D visualization of vascular networks: A review.Vascul Pharmacol. 2021 Dec;141:106905. doi: 10.1016/j.vph.2021.106905. Epub 2021 Sep 20. Vascul Pharmacol. 2021. PMID: 34506969 Review.
-
Recent progress in optical clearing of eye tissues.Exp Eye Res. 2021 Nov;212:108796. doi: 10.1016/j.exer.2021.108796. Epub 2021 Oct 15. Exp Eye Res. 2021. PMID: 34662543 Review.
-
Tissue clearing technique: Recent progress and biomedical applications.J Anat. 2021 Feb;238(2):489-507. doi: 10.1111/joa.13309. Epub 2020 Sep 16. J Anat. 2021. PMID: 32939792 Free PMC article. Review.
Cited by
-
Three-dimensional visualization of cerebral blood vessels and neural changes in thick ischemic rat brain slices using tissue clearing.Sci Rep. 2022 Sep 23;12(1):15897. doi: 10.1038/s41598-022-19575-w. Sci Rep. 2022. PMID: 36151103 Free PMC article.
-
High-Speed Clearing and High-Resolution Staining for Analysis of Various Markers for Neurons and Vessels.Tissue Eng Regen Med. 2024 Oct;21(7):1037-1048. doi: 10.1007/s13770-024-00658-w. Epub 2024 Jul 2. Tissue Eng Regen Med. 2024. PMID: 38955906
-
A 3D Analysis of Cleared Human Melanoma.Biomedicines. 2022 Jul 2;10(7):1580. doi: 10.3390/biomedicines10071580. Biomedicines. 2022. PMID: 35884885 Free PMC article.
-
A Review of Ex Vivo X-ray Microfocus Computed Tomography-Based Characterization of the Cardiovascular System.Int J Mol Sci. 2021 Mar 23;22(6):3263. doi: 10.3390/ijms22063263. Int J Mol Sci. 2021. PMID: 33806852 Free PMC article. Review.
-
A Guide to Examining Intramuscular Fat Formation and its Cellular Origin in Skeletal Muscle.J Vis Exp. 2022 May 26;(183):10.3791/63996. doi: 10.3791/63996. J Vis Exp. 2022. PMID: 35695517 Free PMC article.
References
-
- Abe J., Ozga A.J., Swoger J., Sharpe J., Ripoll J., Stein J.V. Light sheet fluorescence microscopy for in situ cell interaction analysis in mouse lymph nodes. J. Immunol. Methods. 2016;431:1–10. - PubMed
-
- Anzai Y., Minoshima S., Lee V.S. Enhancing value of MRI: a call for action. J. Magn. Reson. Imaging. 2019;49:e40–e48. - PubMed
-
- Arranz A., Dong D., Zhu S., Rudin M., Tsatsanis C., Tian J., Ripoll J. Helical optical projection tomography. Opt. Express. 2013;21:25912–25925. - PubMed
Publication types
LinkOut - more resources
Full Text Sources