Overcoming the Design Challenge in 3D Biomimetic Hybrid Scaffolds for Bone and Osteochondral Regeneration by Factorial Design
- PMID: 32775321
- PMCID: PMC7381347
- DOI: 10.3389/fbioe.2020.00743
Overcoming the Design Challenge in 3D Biomimetic Hybrid Scaffolds for Bone and Osteochondral Regeneration by Factorial Design
Abstract
Scaffolds for bone regeneration have been engineered by a plethora of manufacturing technologies and biomaterials. However, the performance of these systems is often limited by lack of robustness in the process design, that hampers their scalability to clinical application. In the present study, Design of Experiment (DoE) was used as statistical tool to design the biofabrication of hybrid hydroxyapatite (HA)/collagen scaffolds for bone regeneration and optimize their integration in a multilayer osteochondral device. The scaffolds were synthesized via a multi-step bioinspired process consisting in HA nano-crystals nucleation on the collagen self-assembling fibers and ribose glycation was used as collagen cross-linking method to modulate the mechanical and physical properties. The process design was performed by selecting hydrogel concentration, HA/collagen ratio and cross-linker content as key variables and the fabrication was carried out basing on a full factorial design. Scaffold performances were tested by evaluating porosity, swelling ratio, degradation rate and mechanical behavior as model output responses while physicochemical properties of the constructs were evaluated by TGA, ICP, FT-IR spectroscopy, and XRD analysis. Physicochemical characterizations confirmed the nucleation of a biomimetic inorganic phase and the interaction of the HA and collagenic components. The DoE model revealed a significant interaction between HA content and collagen cross-linking in determining porosity, swelling and mechanical properties of the scaffolds. The combined effect of hydrogel concentration and mineral phase played a key role on porosity and swelling while degradation resulted to be mainly affected by the HA loading and ribose content. The model was then used to determine the suitable input parameters for the synthesis of multi-layer scaffolds with graded mineralization rate, that can be used to mimic the whole cartilage-bone interface. This work proved that experimental design applied to complex biofabrication processes represents an effective and reliable way to design hybrid constructs with standardized and tunable properties for osteochondral tissue engineering.
Keywords: biomineralization; collagen cross-linking; factorial design; hybrid scaffold; osteochondral regeneration.
Copyright © 2020 Dellaquila, Campodoni, Tampieri and Sandri.
Figures
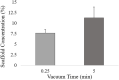
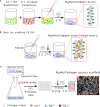
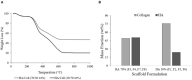
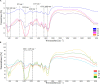
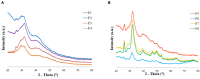
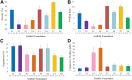
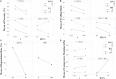
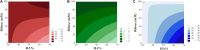
Similar articles
-
Ribose mediated crosslinking of collagen-hydroxyapatite hybrid scaffolds for bone tissue regeneration using biomimetic strategies.Mater Sci Eng C Mater Biol Appl. 2017 Aug 1;77:594-605. doi: 10.1016/j.msec.2017.03.255. Epub 2017 Mar 28. Mater Sci Eng C Mater Biol Appl. 2017. PMID: 28532070
-
Effect of different hydroxyapatite incorporation methods on the structural and biological properties of porous collagen scaffolds for bone repair.J Anat. 2015 Dec;227(6):732-45. doi: 10.1111/joa.12262. Epub 2014 Nov 20. J Anat. 2015. PMID: 25409684 Free PMC article.
-
A new bi-layered scaffold for osteochondral tissue regeneration: In vitro and in vivo preclinical investigations.Mater Sci Eng C Mater Biol Appl. 2017 Jan 1;70(Pt 1):101-111. doi: 10.1016/j.msec.2016.08.027. Epub 2016 Aug 12. Mater Sci Eng C Mater Biol Appl. 2017. PMID: 27770869
-
Porous Scaffolds for Regeneration of Cartilage, Bone and Osteochondral Tissue.Adv Exp Med Biol. 2018;1058:171-191. doi: 10.1007/978-3-319-76711-6_8. Adv Exp Med Biol. 2018. PMID: 29691822 Review.
-
Micro/Nano Scaffolds for Osteochondral Tissue Engineering.Adv Exp Med Biol. 2018;1058:125-139. doi: 10.1007/978-3-319-76711-6_6. Adv Exp Med Biol. 2018. PMID: 29691820 Review.
Cited by
-
Towards the Experimentally-Informed In Silico Nozzle Design Optimization for Extrusion-Based Bioprinting of Shear-Thinning Hydrogels.Front Bioeng Biotechnol. 2021 Aug 6;9:701778. doi: 10.3389/fbioe.2021.701778. eCollection 2021. Front Bioeng Biotechnol. 2021. PMID: 34422780 Free PMC article.
-
Medicated Hydroxyapatite/Collagen Hybrid Scaffolds for Bone Regeneration and Local Antimicrobial Therapy to Prevent Bone Infections.Pharmaceutics. 2021 Jul 16;13(7):1090. doi: 10.3390/pharmaceutics13071090. Pharmaceutics. 2021. PMID: 34371782 Free PMC article.
-
The biological applications of IPN hydrogels.ADMET DMPK. 2024 Aug 14;12(4):581-621. doi: 10.5599/admet.2398. eCollection 2024. ADMET DMPK. 2024. PMID: 39473628 Free PMC article. Review.
-
Robotics-Driven Manufacturing of Cartilaginous Microtissues for Skeletal Tissue Engineering Applications.Stem Cells Transl Med. 2024 Mar 15;13(3):278-292. doi: 10.1093/stcltm/szad091. Stem Cells Transl Med. 2024. PMID: 38217535 Free PMC article.
-
Computational methods for biofabrication in tissue engineering and regenerative medicine - a literature review.Comput Struct Biotechnol J. 2024 Jan 2;23:601-616. doi: 10.1016/j.csbj.2023.12.035. eCollection 2024 Dec. Comput Struct Biotechnol J. 2024. PMID: 38283852 Free PMC article. Review.
References
-
- Bahçecitapar M., Karadağ Ataş Ö., Aktaş Altunay S. (2016). Estimation of sample size and power for general full factorial designs. Ýstatistikçiler Derg. Ýstatistik Aktüerya 9 79–86.
LinkOut - more resources
Full Text Sources