Deletion of a Peptidylprolyl Isomerase Gene Results in the Inability of Caldicellulosiruptor bescii To Grow on Crystalline Cellulose without Affecting Protein Glycosylation or Growth on Soluble Substrates
- PMID: 32769195
- PMCID: PMC7531974
- DOI: 10.1128/AEM.00909-20
Deletion of a Peptidylprolyl Isomerase Gene Results in the Inability of Caldicellulosiruptor bescii To Grow on Crystalline Cellulose without Affecting Protein Glycosylation or Growth on Soluble Substrates
Abstract
Caldicellulosiruptor bescii secretes a large number of complementary multifunctional enzymes with unique activities for biomass deconstruction. The most abundant enzymes in the C. bescii secretome are found in a unique gene cluster containing a glycosyl transferase (GT39) and a putative peptidyl prolyl cis-trans isomerase. Deletion of the glycosyl transferase in this cluster resulted in loss of detectable protein glycosylation in C. bescii, and its activity has been shown to be responsible for the glycosylation of the proline-threonine rich linkers found in many of the multifunctional cellulases. The presence of a putative peptidyl prolyl cis-trans isomerase within this gene cluster suggested that it might also play a role in cellulase modification. Here, we identify this gene as a putative prsA prolyl cis-trans isomerase. Deletion of prsA2 leads to the inability of C. bescii to grow on insoluble substrates such as Avicel, the model cellulose substrate, while exhibiting no differences in phenotype with the wild-type strain on soluble substrates. Finally, we provide evidence that the prsA2 gene is likely needed to increase solubility of multifunctional cellulases and that this unique gene cluster was likely acquired by members of the Caldicellulosiruptor genus with a group of genes to optimize the production and activity of multifunctional cellulases.IMPORTANCECaldicellulosiruptor has the ability to digest complex plant biomass without pretreatment and have been engineered to convert biomass, a sustainable, carbon neutral substrate, to fuels. Their strategy for deconstructing plant cell walls relies on an interesting class of cellulases consisting of multiple catalytic modules connected by linker regions and carbohydrate binding modules. The best studied of these enzymes, CelA, has a unique deconstruction mechanism. CelA is located in a cluster of genes that likely allows for optimal expression, secretion, and activity. One of the genes in this cluster is a putative isomerase that modifies the CelA protein. In higher eukaryotes, these isomerases are essential for the proper folding of glycoproteins in the endoplasmic reticulum, but little is known about the role of isomerization in cellulase activity. We show that the stability and activity of CelA is dependent on the activity of this isomerase.
Keywords: biomass deconstruction; cellulase; prolyl isomerases.
Copyright © 2020 American Society for Microbiology.
Figures
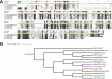
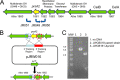
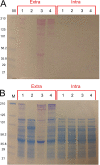
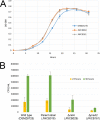
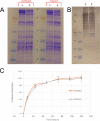
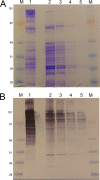
Similar articles
-
Functional Analysis of the Glucan Degradation Locus in Caldicellulosiruptor bescii Reveals Essential Roles of Component Glycoside Hydrolases in Plant Biomass Deconstruction.Appl Environ Microbiol. 2017 Dec 1;83(24):e01828-17. doi: 10.1128/AEM.01828-17. Print 2017 Dec 15. Appl Environ Microbiol. 2017. PMID: 28986379 Free PMC article.
-
Expression of a Cellobiose Phosphorylase from Thermotoga maritima in Caldicellulosiruptor bescii Improves the Phosphorolytic Pathway and Results in a Dramatic Increase in Cellulolytic Activity.Appl Environ Microbiol. 2018 Jan 17;84(3):e02348-17. doi: 10.1128/AEM.02348-17. Print 2018 Feb 1. Appl Environ Microbiol. 2018. PMID: 29101202 Free PMC article.
-
Homologous expression of the Caldicellulosiruptor bescii CelA reveals that the extracellular protein is glycosylated.PLoS One. 2015 Mar 23;10(3):e0119508. doi: 10.1371/journal.pone.0119508. eCollection 2015. PLoS One. 2015. PMID: 25799047 Free PMC article.
-
Computational perspective and evaluation of plausible catalytic mechanisms of peptidyl-prolyl cis-trans isomerases.Biochim Biophys Acta. 2015 Oct;1850(10):1994-2004. doi: 10.1016/j.bbagen.2014.12.023. Epub 2015 Jan 10. Biochim Biophys Acta. 2015. PMID: 25585011 Review.
-
Prolyl cis-trans isomerization as a molecular timer.Nat Chem Biol. 2007 Oct;3(10):619-29. doi: 10.1038/nchembio.2007.35. Nat Chem Biol. 2007. PMID: 17876319 Review.
References
-
- Yang SJ, Kataeva I, Hamilton-Brehm SD, Engle NL, Tschaplinski TJ, Doeppke C, Davis M, Westpheling J, Adams MW. 2009. Efficient degradation of lignocellulosic plant biomass, without pretreatment, by the thermophilic anaerobe “Anaerocellum thermophilum” DSM 6725. Appl Environ Microbiol 75:4762–4769. doi:10.1128/AEM.00236-09. - DOI - PMC - PubMed
-
- Blumer-Schuette SE, Giannone RJ, Zurawski JV, Ozdemir I, Ma Q, Yin Y, Xu Y, Kataeva I, Poole FL II, Adams MW, Hamilton-Brehm SD, Elkins JG, Larimer FW, Land ML, Hauser LJ, Cottingham RW, Hettich RL, Kelly RM. 2012. Caldicellulosiruptor core and pangenomes reveal determinants for noncellulosomal thermophilic deconstruction of plant biomass. J Bacteriol 194:4015–4028. doi:10.1128/JB.00266-12. - DOI - PMC - PubMed
-
- Lee LL, Blumer-Schuette SE, Izquierdo JA, Zurawski JV, Loder AJ, Conway JM, Elkins JG, Podar M, Clum A, Jones PC, Piatek MJ, Weighill DA, Jacobson DA, Adams MWW, Kelly RM. 2018. Genus-wide assessment of lignocellulose utilization in the extremely thermophilic genus Caldicellulosiruptor by genomic, pangenomic, and metagenomic analyses. Appl Environ Microbiol 84:e02694-17. doi:10.1128/AEM.02694-17. - DOI - PMC - PubMed
-
- Brunecky R, Alahuhta M, Xu Q, Donohoe BS, Crowley MF, Kataeva IA, Yang SJ, Resch MG, Adams MW, Lunin VV, Himmel ME, Bomble YJ. 2013. Revealing nature’s cellulase diversity: the digestion mechanism of Caldicellulosiruptor bescii CelA. Science 342:1513–1516. doi:10.1126/science.1244273. - DOI - PubMed
Publication types
MeSH terms
Substances
Supplementary concepts
LinkOut - more resources
Full Text Sources