Mouse Astrocytes Promote Microglial Ramification by Releasing TGF-β and Forming Glial Fibers
- PMID: 32754014
- PMCID: PMC7366495
- DOI: 10.3389/fncel.2020.00195
Mouse Astrocytes Promote Microglial Ramification by Releasing TGF-β and Forming Glial Fibers
Abstract
The morphology of microglial cells is often closely related to their functions. The mechanisms that regulate microglial ramification are not well understood. Here we reveal the biological mechanisms by which astrocytes regulate microglial ramification. Morphological variation in mouse microglial cultures was measured in terms of cell area as well as branch number and length. Effects on microglial ramification were analyzed after microinjecting the toxin L-alpha-aminoadipic acid (L-AAA) in the mouse cortex or hippocampus to ablate astrocytes, and after culturing microglia on their own in an astrocyte-conditioned medium (ACM) or together with astrocytes in coculture. TGF-β expression was determined by Western blotting, immunohistochemistry, and ELISA. The TGF-β signaling pathway was blocked by the TGF-β antibody to assess the role of TGF-β on microglial ramification. The results showed that microglia had more and longer branches and smaller cell bodies in brain areas where astrocytes were abundant. In the mouse cortex and hippocampus, ablation of astrocytes by L-AAA decreased number and length of microglial branches and increased the size of cell bodies. Similar results were obtained with isolated microglia in culture. However, isolated microglia were able to maintain their multibranched structure for a long time when cultured on astrocyte monolayers. Ameboid microglia isolated from P0 to P3 mice showed increased ramification when cultured in ACM or on astrocyte monolayers. Microglia cultured on astrocyte monolayers showed more complex branching structures than those cultured in ACM. Blocking astrocyte-derived TGF-β decreased microglial ramification. Astrocytes induced the formation of protuberances on branches of microglia by forming glial fibers that increased traction. These experiments in mice suggest that astrocytes promote microglial ramification by forming glial fibers to create traction and by secreting soluble factors into the surroundings. For example, astrocyte-secreted TGF-β promotes microglia to generate primitive branches, whose ramification is refined by glial fibers.
Keywords: TGF-β; astrocyte; glial fibrillary acidic protein; microglia; microglial ramification.
Copyright © 2020 Zhang, Zhang, Yi, Jiang, Qiao, Zhang, Xiao and Zhou.
Figures
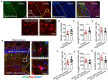
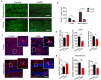
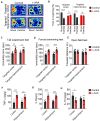
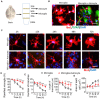
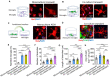
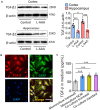
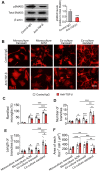
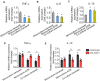
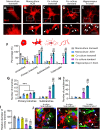
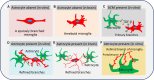
Similar articles
-
Astrocyte-released cytokines induce ramification and outward K+ channel expression in microglia via distinct signalling pathways.Eur J Neurosci. 2001 Aug;14(3):463-73. doi: 10.1046/j.0953-816x.2001.01661.x. Eur J Neurosci. 2001. PMID: 11553296
-
Microglial ramification requires nondiffusible factors derived from astrocytes.Exp Neurol. 1996 Feb;137(2):367-75. doi: 10.1006/exnr.1996.0038. Exp Neurol. 1996. PMID: 8635553
-
Macrophage colony-stimulating factor mediates astrocyte-induced microglial ramification in human fetal central nervous system culture.Am J Pathol. 1994 Jul;145(1):48-53. Am J Pathol. 1994. PMID: 8030755 Free PMC article.
-
An Overview on the Differential Interplay Among Neurons-Astrocytes-Microglia in CA1 and CA3 Hippocampus in Hypoxia/Ischemia.Front Cell Neurosci. 2020 Nov 11;14:585833. doi: 10.3389/fncel.2020.585833. eCollection 2020. Front Cell Neurosci. 2020. PMID: 33262692 Free PMC article. Review.
-
Cellular and molecular correlates to plasticity during recovery from injury in the developing mammalian brain.Prog Brain Res. 1996;108:365-77. doi: 10.1016/s0079-6123(08)62552-2. Prog Brain Res. 1996. PMID: 8979814 Review.
Cited by
-
Human iPSC-derived glia models for the study of neuroinflammation.J Neuroinflammation. 2023 Oct 10;20(1):231. doi: 10.1186/s12974-023-02919-2. J Neuroinflammation. 2023. PMID: 37817184 Free PMC article. Review.
-
Antidepressant Effect of Heracleum moellendorffii Extract on Behavioral Changes in Astrocyte Ablation Mouse Model of Depression by Modulating Neuroinflammation through the Inhibition of Lipocalin-2.Nutrients. 2024 Jun 27;16(13):2049. doi: 10.3390/nu16132049. Nutrients. 2024. PMID: 38999797 Free PMC article.
-
Increasing Severity of Spinal Cord Injury Results in Microglia/Macrophages With Annular-Shaped Morphology and No Change in Expression of CD40 and Tumor Growth Factor-β During the Chronic Post-injury Stage.Front Mol Neurosci. 2022 Feb 24;14:802558. doi: 10.3389/fnmol.2021.802558. eCollection 2021. Front Mol Neurosci. 2022. PMID: 35282656 Free PMC article.
-
Mapping the Plasticity of Morphology, Molecular Properties and Function in Mouse Primary Microglia.Front Cell Neurosci. 2022 Jan 26;15:811061. doi: 10.3389/fncel.2021.811061. eCollection 2021. Front Cell Neurosci. 2022. PMID: 35153675 Free PMC article.
-
Targeting astrocytes polarization after spinal cord injury: a promising direction.Front Cell Neurosci. 2024 Oct 16;18:1478741. doi: 10.3389/fncel.2024.1478741. eCollection 2024. Front Cell Neurosci. 2024. PMID: 39479524 Free PMC article. Review.
References
LinkOut - more resources
Full Text Sources
Other Literature Sources
Research Materials