3D printing of gas-dynamic virtual nozzles and optical characterization of high-speed microjets
- PMID: 32752448
- PMCID: PMC7470680
- DOI: 10.1364/OE.390131
3D printing of gas-dynamic virtual nozzles and optical characterization of high-speed microjets
Abstract
Gas dynamic virtual nozzles (GDVNs) produce microscopic flow-focused liquid jets and droplets and play an important role at X-ray free-electron laser (XFEL) facilities where they are used to steer a stream of hydrated biomolecules into an X-ray focus during diffraction measurements. Highly stable and reproducible microjet and microdroplets are desired, as are flexible fabrication methods that enable integrated mixing microfluidics, droplet triggering mechanisms, laser illumination, and other customized features. In this study, we develop the use of high-resolution 3D nano-printing for the production of monolithic, asymmetric GDVN designs that are difficult to fabricate by other means. We also develop a dual-pulsed nanosecond image acquisition and analysis platform for the characterization of GDVN performance, including jet speed, length, diameter, and directionality, among others. We show that printed GDVNs can form microjets with very high degree of reproducibility, down to sub-micron diameters, and with water jet speeds beyond 170 m/s.
Conflict of interest statement
The authors declare no conflicts of interest.
Figures
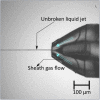
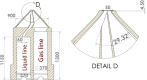
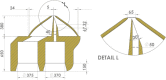
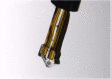
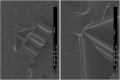
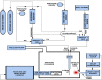
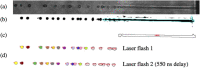
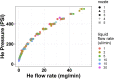
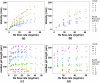
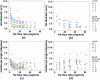
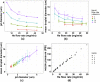
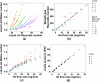
Similar articles
-
3D printed devices and infrastructure for liquid sample delivery at the European XFEL.J Synchrotron Radiat. 2022 Mar 1;29(Pt 2):331-346. doi: 10.1107/S1600577521013370. Epub 2022 Feb 15. J Synchrotron Radiat. 2022. PMID: 35254295 Free PMC article.
-
Recent Advances and Future Perspectives on Microfluidic Mix-and-Jet Sample Delivery Devices.Micromachines (Basel). 2021 May 7;12(5):531. doi: 10.3390/mi12050531. Micromachines (Basel). 2021. PMID: 34067131 Free PMC article. Review.
-
Three-dimensional-printed gas dynamic virtual nozzles for x-ray laser sample delivery.Opt Express. 2016 May 30;24(11):11515-30. doi: 10.1364/OE.24.011515. Opt Express. 2016. PMID: 27410079 Free PMC article.
-
Microfluidic polyimide gas dynamic virtual nozzles for serial crystallography.Rev Sci Instrum. 2020 Aug 1;91(8):085108. doi: 10.1063/5.0012806. Rev Sci Instrum. 2020. PMID: 32872940
-
The emerging role of microfluidics in multi-material 3D bioprinting.Lab Chip. 2020 Jun 21;20(12):2044-2056. doi: 10.1039/c9lc01184f. Epub 2020 May 27. Lab Chip. 2020. PMID: 32459222 Review.
Cited by
-
Electrically stimulated droplet injector for reduced sample consumption in serial crystallography.Biophys Rep (N Y). 2022 Sep 29;2(4):100081. doi: 10.1016/j.bpr.2022.100081. eCollection 2022 Dec 14. Biophys Rep (N Y). 2022. PMID: 36425668 Free PMC article.
-
3D printed devices and infrastructure for liquid sample delivery at the European XFEL.J Synchrotron Radiat. 2022 Mar 1;29(Pt 2):331-346. doi: 10.1107/S1600577521013370. Epub 2022 Feb 15. J Synchrotron Radiat. 2022. PMID: 35254295 Free PMC article.
-
Co-flow injection for serial crystallography at X-ray free-electron lasers.J Appl Crystallogr. 2022 Feb 1;55(Pt 1):1-13. doi: 10.1107/S1600576721011079. eCollection 2022 Feb 1. J Appl Crystallogr. 2022. PMID: 35153640 Free PMC article.
-
The XBI BioLab for life science experiments at the European XFEL.J Appl Crystallogr. 2021 Feb 1;54(Pt 1):7-21. doi: 10.1107/S1600576720013989. eCollection 2021 Feb 1. J Appl Crystallogr. 2021. PMID: 33833637 Free PMC article.
-
Recent Advances and Future Perspectives on Microfluidic Mix-and-Jet Sample Delivery Devices.Micromachines (Basel). 2021 May 7;12(5):531. doi: 10.3390/mi12050531. Micromachines (Basel). 2021. PMID: 34067131 Free PMC article. Review.
References
-
- Gañán-Calvo A. M., “Generation of steady liquid microthreads and micron-sized monodisperse sprays in gas streams,” Phys. Rev. Lett. 80(2), 285–288 (1998).10.1103/PhysRevLett.80.285 - DOI
-
- Stan C. A., Milathianaki D., Laksmono H., Sierra R. G., McQueen T. A., Messerschmidt M., Williams G. J., Koglin J. E., Lane T. J., Hayes M. J., Hayes M. J., Guillet S. A. H., Liang M., Aquila A. L., Willmott P. R., Robinson J. S., Gumerlock K. L., Botha S., Nass K., Schlichting I., Shoeman R. L., Stone H. A., Boutet S., “Liquid explosions induced by x-ray laser pulses,” Nat. Phys. 12(10), 966–971 (2016).10.1038/nphys3779 - DOI
Grants and funding
LinkOut - more resources
Full Text Sources