Structural stability of SARS-CoV-2 3CLpro and identification of quercetin as an inhibitor by experimental screening
- PMID: 32745548
- PMCID: PMC7395220
- DOI: 10.1016/j.ijbiomac.2020.07.235
Structural stability of SARS-CoV-2 3CLpro and identification of quercetin as an inhibitor by experimental screening
Abstract
The global health emergency generated by coronavirus disease 2019 (COVID-19) has prompted the search for preventive and therapeutic treatments for its pathogen, the severe acute respiratory syndrome coronavirus 2 (SARS-CoV-2). There are many potential targets for drug discovery and development to tackle this disease. One of these targets is the main protease, Mpro or 3CLpro, which is highly conserved among coronaviruses. 3CLpro is an essential player in the viral replication cycle, processing the large viral polyproteins and rendering the individual proteins functional. We report a biophysical characterization of the structural stability and the catalytic activity of 3CLpro from SARS-CoV-2, from which a suitable experimental in vitro molecular screening procedure has been designed. By screening of a small chemical library consisting of about 150 compounds, the natural product quercetin was identified as reasonably potent inhibitor of SARS-CoV-2 3CLpro (Ki ~ 7 μM). Quercetin could be shown to interact with 3CLpro using biophysical techniques and bind to the active site in molecular simulations. Quercetin, with well-known pharmacokinetic and ADMET properties, can be considered as a good candidate for further optimization and development, or repositioned for COVID-19 therapeutic treatment.
Keywords: Drug discovery; SARS-CoV-2 3CL protease; Structural stability.
Copyright © 2020 Elsevier B.V. All rights reserved.
Conflict of interest statement
Declaration of competing interest The authors declare no conflict of interest.
Figures
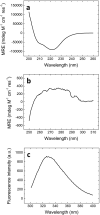
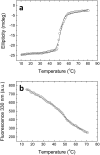
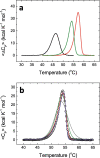
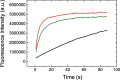
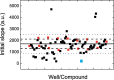
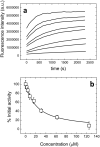
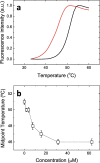
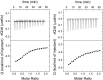
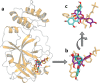
Similar articles
-
Targeting the Dimerization of the Main Protease of Coronaviruses: A Potential Broad-Spectrum Therapeutic Strategy.ACS Comb Sci. 2020 Jun 8;22(6):297-305. doi: 10.1021/acscombsci.0c00058. Epub 2020 May 27. ACS Comb Sci. 2020. PMID: 32402186 Review.
-
Optimization Rules for SARS-CoV-2 Mpro Antivirals: Ensemble Docking and Exploration of the Coronavirus Protease Active Site.Viruses. 2020 Aug 26;12(9):942. doi: 10.3390/v12090942. Viruses. 2020. PMID: 32859008 Free PMC article.
-
Identification of high-affinity inhibitors of SARS-CoV-2 main protease: Towards the development of effective COVID-19 therapy.Virus Res. 2020 Oct 15;288:198102. doi: 10.1016/j.virusres.2020.198102. Epub 2020 Jul 24. Virus Res. 2020. PMID: 32717346 Free PMC article.
-
Drug Repurposing for Candidate SARS-CoV-2 Main Protease Inhibitors by a Novel In Silico Method.Molecules. 2020 Aug 23;25(17):3830. doi: 10.3390/molecules25173830. Molecules. 2020. PMID: 32842509 Free PMC article.
-
Design and Evaluation of Anti-SARS-Coronavirus Agents Based on Molecular Interactions with the Viral Protease.Molecules. 2020 Aug 27;25(17):3920. doi: 10.3390/molecules25173920. Molecules. 2020. PMID: 32867349 Free PMC article. Review.
Cited by
-
A Blueprint for High Affinity SARS-CoV-2 Mpro Inhibitors from Activity-Based Compound Library Screening Guided by Analysis of Protein Dynamics.ACS Pharmacol Transl Sci. 2021 Mar 16;4(3):1079-1095. doi: 10.1021/acsptsci.0c00215. eCollection 2021 Jun 11. ACS Pharmacol Transl Sci. 2021. PMID: 34136757 Free PMC article.
-
A critical evaluation of risk to reward ratio of quercetin supplementation for COVID-19 and associated comorbid conditions.Phytother Res. 2022 Jun;36(6):2394-2415. doi: 10.1002/ptr.7461. Epub 2022 Apr 8. Phytother Res. 2022. PMID: 35393674 Free PMC article. Review.
-
Accelerating the repurposing of FDA-approved drugs against coronavirus disease-19 (COVID-19).RSC Adv. 2020 Nov 10;10(67):40867-40875. doi: 10.1039/d0ra09010g. eCollection 2020 Nov 9. RSC Adv. 2020. PMID: 35519188 Free PMC article.
-
Molecular Mechanisms of Possible Action of Phenolic Compounds in COVID-19 Protection and Prevention.Int J Mol Sci. 2021 Nov 17;22(22):12385. doi: 10.3390/ijms222212385. Int J Mol Sci. 2021. PMID: 34830267 Free PMC article. Review.
-
Recent advances towards natural plants as potential inhibitors of SARS-Cov-2 targets.Pharm Biol. 2023 Dec;61(1):1186-1210. doi: 10.1080/13880209.2023.2241518. Pharm Biol. 2023. PMID: 37605622 Free PMC article. Review.
References
-
- W.H.O. (WHO) World Health Organization. Novel coronavirus (COVID-19) situation. https://covid19.who.int - PMC - PubMed
-
- Huang C., Wang Y., Li X., Ren L., Zhao J., Hu Y., Zhang L., Fan G., Xu J., Gu X., Cheng Z., Yu T., Xia J., Wei Y., Wu W., Xie X., Yin W., Li H., Liu M., Xiao Y., Gao H., Guo L., Xie J., Wang G., Jiang R., Gao Z., Jin Q., Wang J., Cao B. Clinical features of patients infected with 2019 novel coronavirus in Wuhan, China. Lancet. 2020;395(10223):497–506. - PMC - PubMed
-
- Adams J.G., Walls R.M. JAMA; United States: 2020. Supporting the Health Care Workforce During the COVID-19 Global Epidemic. - PubMed
MeSH terms
Substances
LinkOut - more resources
Full Text Sources
Other Literature Sources
Miscellaneous