EGFR forms ligand-independent oligomers that are distinct from the active state
- PMID: 32727847
- PMCID: PMC7504936
- DOI: 10.1074/jbc.RA120.012852
EGFR forms ligand-independent oligomers that are distinct from the active state
Abstract
The human epidermal growth factor receptor (EGFR/ERBB1) is a receptor tyrosine kinase (RTK) that forms activated oligomers in response to ligand. Much evidence indicates that EGFR/ERBB1 also forms oligomers in the absence of ligand, but the structure and physiological role of these ligand-independent oligomers remain unclear. To examine these features, we use fluorescence microscopy to measure the oligomer stability and FRET efficiency for homo- and hetero-oligomers of fluorescent protein-labeled forms of EGFR and its paralog, human epidermal growth factor receptor 2 (HER2/ERBB2) in vesicles derived from mammalian cell membranes. We observe that both receptors form ligand-independent oligomers at physiological plasma membrane concentrations. Mutations introduced in the kinase region at the active state asymmetric kinase dimer interface do not affect the stability of ligand-independent EGFR oligomers. These results indicate that ligand-independent EGFR oligomers form using interactions that are distinct from the EGFR active state.
Keywords: EGFR; FRET; epidermal growth factor receptor; fluorescence resonance energy transfer; membrane protein; oligomerization; receptor tyrosine kinase; signal transduction.
© 2020 Byrne et al.
Conflict of interest statement
Conflict of interest—The authors declare that they have no conflicts of interest with the contents of this article.
Figures
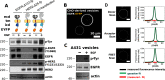
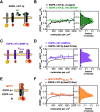
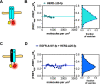
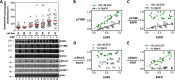
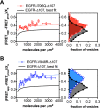
Similar articles
-
EGFR oligomerization organizes kinase-active dimers into competent signalling platforms.Nat Commun. 2016 Oct 31;7:13307. doi: 10.1038/ncomms13307. Nat Commun. 2016. PMID: 27796308 Free PMC article.
-
Quantitation of the effect of ErbB2 on epidermal growth factor receptor binding and dimerization.J Biol Chem. 2012 Sep 7;287(37):31116-25. doi: 10.1074/jbc.M112.373647. Epub 2012 Jul 20. J Biol Chem. 2012. PMID: 22822073 Free PMC article.
-
Distribution of resting and ligand-bound ErbB1 and ErbB2 receptor tyrosine kinases in living cells using number and brightness analysis.Proc Natl Acad Sci U S A. 2010 Sep 21;107(38):16524-9. doi: 10.1073/pnas.1002642107. Epub 2010 Sep 2. Proc Natl Acad Sci U S A. 2010. PMID: 20813958 Free PMC article.
-
ErbB Receptors and Cancer.Methods Mol Biol. 2017;1652:3-35. doi: 10.1007/978-1-4939-7219-7_1. Methods Mol Biol. 2017. PMID: 28791631 Review.
-
Small molecule inhibitors targeting the EGFR/ErbB family of protein-tyrosine kinases in human cancers.Pharmacol Res. 2019 Jan;139:395-411. doi: 10.1016/j.phrs.2018.11.014. Epub 2018 Nov 27. Pharmacol Res. 2019. PMID: 30500458 Review.
Cited by
-
Targeted Inhibitors of EGFR: Structure, Biology, Biomarkers, and Clinical Applications.Cells. 2023 Dec 25;13(1):47. doi: 10.3390/cells13010047. Cells. 2023. PMID: 38201251 Free PMC article. Review.
-
Fluorescence Imaging of Epidermal Growth Factor Receptor Tyrosine Kinase Inhibitor Resistance in Non-Small Cell Lung Cancer.Cancers (Basel). 2022 Jan 28;14(3):686. doi: 10.3390/cancers14030686. Cancers (Basel). 2022. PMID: 35158954 Free PMC article. Review.
-
Mechanical disruption of E-cadherin complexes with epidermal growth factor receptor actuates growth factor-dependent signaling.Proc Natl Acad Sci U S A. 2022 Jan 25;119(4):e2100679119. doi: 10.1073/pnas.2100679119. Proc Natl Acad Sci U S A. 2022. PMID: 35074920 Free PMC article.
-
Axl contributes to efficient migration and invasion of melanoma cells.PLoS One. 2023 Mar 29;18(3):e0283749. doi: 10.1371/journal.pone.0283749. eCollection 2023. PLoS One. 2023. PMID: 36989239 Free PMC article.
-
A cancer mutation promotes EphA4 oligomerization and signaling by altering the conformation of the SAM domain.J Biol Chem. 2021 Jul;297(1):100876. doi: 10.1016/j.jbc.2021.100876. Epub 2021 Jun 15. J Biol Chem. 2021. PMID: 34139238 Free PMC article.
References
Publication types
MeSH terms
Substances
Grants and funding
LinkOut - more resources
Full Text Sources
Research Materials
Miscellaneous