Strain-Specific Epigenetic Regulation of Endogenous Retroviruses: The Role of Trans-Acting Modifiers
- PMID: 32727076
- PMCID: PMC7472028
- DOI: 10.3390/v12080810
Strain-Specific Epigenetic Regulation of Endogenous Retroviruses: The Role of Trans-Acting Modifiers
Abstract
Approximately 10 percent of the mouse genome consists of endogenous retroviruses (ERVs), relics of ancient retroviral infections that are classified based on their relatedness to exogenous retroviral genera. Because of the ability of ERVs to retrotranspose, as well as their cis-acting regulatory potential due to functional elements located within the elements, mammalian ERVs are generally subject to epigenetic silencing by DNA methylation and repressive histone modifications. The mobilisation and expansion of ERV elements is strain-specific, leading to ERVs being highly polymorphic between inbred mouse strains, hinting at the possibility of the strain-specific regulation of ERVs. In this review, we describe the existing evidence of mouse strain-specific epigenetic control of ERVs and discuss the implications of differential ERV regulation on epigenetic inheritance models. We consider Krüppel-associated box domain (KRAB) zinc finger proteins as likely candidates for strain-specific ERV modifiers, drawing on insights gained from the study of the strain-specific behaviour of transgenes. We conclude by considering the coevolution of KRAB zinc finger proteins and actively transposing ERV elements, and highlight the importance of cross-strain studies in elucidating the mechanisms and consequences of strain-specific ERV regulation.
Keywords: ERVs; KRAB zinc finger proteins; epigenetic regulation; metastable epialleles; modifiers; strain-specific; transgenes.
Conflict of interest statement
The authors declare no conflicts of interest associated with this manuscript.
Figures
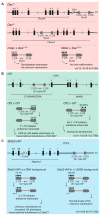
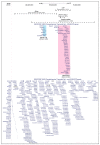
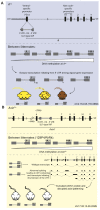
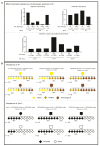
Similar articles
-
The KRAB zinc finger protein ZFP809 is required to initiate epigenetic silencing of endogenous retroviruses.Genes Dev. 2015 Mar 1;29(5):538-54. doi: 10.1101/gad.252767.114. Genes Dev. 2015. PMID: 25737282 Free PMC article.
-
The diversity of zinc-finger genes on human chromosome 19 provides an evolutionary mechanism for defense against inherited endogenous retroviruses.Cell Death Differ. 2014 Mar;21(3):381-7. doi: 10.1038/cdd.2013.150. Epub 2013 Oct 25. Cell Death Differ. 2014. PMID: 24162661 Free PMC article.
-
Epigenetic interplay between mouse endogenous retroviruses and host genes.Genome Biol. 2012 Oct 3;13(10):R89. doi: 10.1186/gb-2012-13-10-r89. Genome Biol. 2012. PMID: 23034137 Free PMC article.
-
Transcription of Endogenous Retroviruses: Broad and Precise Mechanisms of Control.Viruses. 2024 Aug 17;16(8):1312. doi: 10.3390/v16081312. Viruses. 2024. PMID: 39205286 Free PMC article. Review.
-
Silencing and Transcriptional Regulation of Endogenous Retroviruses: An Overview.Viruses. 2020 Aug 13;12(8):884. doi: 10.3390/v12080884. Viruses. 2020. PMID: 32823517 Free PMC article. Review.
Cited by
-
Roles of transposable elements in the regulation of mammalian transcription.Nat Rev Mol Cell Biol. 2022 Jul;23(7):481-497. doi: 10.1038/s41580-022-00457-y. Epub 2022 Feb 28. Nat Rev Mol Cell Biol. 2022. PMID: 35228718 Free PMC article. Review.
-
Analysis of hepatic lentiviral vector transduction; implications for preclinical studies and clinical gene therapy protocols.bioRxiv [Preprint]. 2024 Aug 31:2024.08.20.608805. doi: 10.1101/2024.08.20.608805. bioRxiv. 2024. PMID: 39229157 Free PMC article. Preprint.
-
A spontaneous genetically induced epiallele at a retrotransposon shapes host genome function.Elife. 2021 Mar 23;10:e65233. doi: 10.7554/eLife.65233. Elife. 2021. PMID: 33755012 Free PMC article.
-
Editorial Overview: Endogenous Retroviruses in Development and Disease.Viruses. 2020 Dec 16;12(12):1446. doi: 10.3390/v12121446. Viruses. 2020. PMID: 33339171 Free PMC article.
-
KRAB zinc finger protein diversification drives mammalian interindividual methylation variability.Proc Natl Acad Sci U S A. 2020 Dec 8;117(49):31290-31300. doi: 10.1073/pnas.2017053117. Epub 2020 Nov 25. Proc Natl Acad Sci U S A. 2020. PMID: 33239447 Free PMC article.
References
-
- Boeke J., Stoye J. Retrotransposons, Endogenous Retroviruses, and the Evolution of Retroelements. In: Coffin J.M., Hughes S.H., Varmus H.E., editors. Retroviruses. Volume 1. Cold Spring Harbor Laboratory Press; Cold Spring Harbor, NY, USA: 1997. pp. 123–456. - PubMed
Publication types
MeSH terms
Substances
Grants and funding
LinkOut - more resources
Full Text Sources