Epigenetics in modulating immune functions of stromal and immune cells in the tumor microenvironment
- PMID: 32699350
- PMCID: PMC7609272
- DOI: 10.1038/s41423-020-0505-9
Epigenetics in modulating immune functions of stromal and immune cells in the tumor microenvironment
Abstract
Epigenetic regulation of gene expression in cancer cells has been extensively studied in recent decades, resulting in the FDA approval of multiple epigenetic agents for treating different cancer types. Recent studies have revealed novel roles of epigenetic dysregulation in altering the phenotypes of immune cells and tumor-associated stromal cells, including fibroblasts and endothelial cells. As a result, epigenetic dysregulation of these cells reshapes the tumor microenvironment (TME), changing it from an antitumor environment to an immunosuppressive environment. Here, we review recent studies demonstrating how specific epigenetic mechanisms drive aspects of stromal and immune cell differentiation with implications for the development of solid tumor therapeutics, focusing on the pancreatic ductal adenocarcinoma (PDA) TME as a representative of solid tumors. Due to their unique ability to reprogram the TME into a more immunopermissive environment, epigenetic agents have great potential for sensitizing cancer immunotherapy to augment the antitumor response, as an immunopermissive TME is a prerequisite for the success of cancer immunotherapy but is often not developed with solid tumors. The idea of combining epigenetic agents with cancer immunotherapy has been tested both in preclinical settings and in multiple clinical trials. In this review, we highlight the basic biological mechanisms underlying the synergy between epigenetic therapy and immunotherapy and discuss current efforts to translate this knowledge into clinical benefits for patients.
Keywords: Epigenetics; Immuno-modulation; Tumor microenvironment.
Conflict of interest statement
L.Z. receives grant support from Bristol-Myers Squibb, Merck, iTeos, Amgen, NovaRock, InxMed, and Halozyme. L.Z. is a paid consultant/Advisory Board Member at Biosion, Alphamab, NovaRock, Akrevia, DataRevive, and Mingruzhiyao. L.Z. holds shares of Alphamab and Mingruzhiyao.
Figures
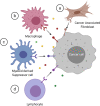
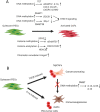
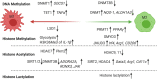
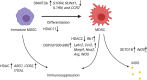
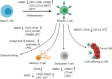
Similar articles
-
Epigenetic control of the tumor microenvironment.Epigenomics. 2016 Dec;8(12):1671-1687. doi: 10.2217/epi-2016-0110. Epub 2016 Oct 4. Epigenomics. 2016. PMID: 27700179 Free PMC article. Review.
-
Epigenetic regulation in the tumor microenvironment: molecular mechanisms and therapeutic targets.Signal Transduct Target Ther. 2023 May 22;8(1):210. doi: 10.1038/s41392-023-01480-x. Signal Transduct Target Ther. 2023. PMID: 37217462 Free PMC article. Review.
-
Epigenetic modulation of antitumor immunity for improved cancer immunotherapy.Mol Cancer. 2021 Dec 20;20(1):171. doi: 10.1186/s12943-021-01464-x. Mol Cancer. 2021. PMID: 34930302 Free PMC article. Review.
-
DNA methylation in the tumor microenvironment.J Zhejiang Univ Sci B. 2017 May;18(5):365-372. doi: 10.1631/jzus.B1600579. J Zhejiang Univ Sci B. 2017. PMID: 28471108 Free PMC article. Review.
-
Intimate communications within the tumor microenvironment: stromal factors function as an orchestra.J Biomed Sci. 2023 Jan 4;30(1):1. doi: 10.1186/s12929-022-00894-z. J Biomed Sci. 2023. PMID: 36600243 Free PMC article. Review.
Cited by
-
Link between m6A modification and infiltration characterization of tumor microenvironment in lung adenocarcinoma.Exp Biol Med (Maywood). 2023 Dec;248(23):2273-2288. doi: 10.1177/15353702231214266. Epub 2024 Jan 2. Exp Biol Med (Maywood). 2023. PMID: 38166412 Free PMC article.
-
Myeloid-derived suppressor cells (MDSC): When good intentions go awry.Cell Immunol. 2021 Apr;362:104302. doi: 10.1016/j.cellimm.2021.104302. Epub 2021 Feb 4. Cell Immunol. 2021. PMID: 33592540 Free PMC article. Review.
-
Autophagy related DNA methylation signature predict clinical prognosis and immune microenvironment in low-grade glioma.Transl Cancer Res. 2022 Jul;11(7):2157-2174. doi: 10.21037/tcr-22-310. Transl Cancer Res. 2022. PMID: 35966301 Free PMC article.
-
M2 Macrophage Co-Expression Factors Correlate With Immune Phenotype and Predict Prognosis of Bladder Cancer.Front Oncol. 2021 Mar 22;11:609334. doi: 10.3389/fonc.2021.609334. eCollection 2021. Front Oncol. 2021. PMID: 33828973 Free PMC article.
-
Enhancing anti-tumor efficacy and immune memory by combining 3p-GPC-3 siRNA treatment with PD-1 blockade in hepatocellular carcinoma.Oncoimmunology. 2022 Jan 2;11(1):2010894. doi: 10.1080/2162402X.2021.2010894. eCollection 2022. Oncoimmunology. 2022. PMID: 36524206 Free PMC article.
References
-
- Rahib L, et al. Projecting cancer incidence and deaths to 2030: the unexpected burden of thyroid, liver, and pancreas cancers in the United States. Cancer Res. 2014;74:2913–2921. - PubMed
-
- Siegel RL, Miller KD, Jemal A. Cancer statistics, 2019. CA Cancer J. Clin. 2019;69:7–34. - PubMed
-
- Ducreux M, et al. Cancer of the pancreas: ESMO Clinical Practice Guidelines for diagnosis, treatment and follow-up. Ann. Oncol. 2015;26(Suppl 5):v56–v68. - PubMed
Publication types
MeSH terms
Grants and funding
LinkOut - more resources
Full Text Sources
Research Materials