α-synuclein strains that cause distinct pathologies differentially inhibit proteasome
- PMID: 32697196
- PMCID: PMC7406352
- DOI: 10.7554/eLife.56825
α-synuclein strains that cause distinct pathologies differentially inhibit proteasome
Abstract
Abnormal α-synuclein aggregation has been implicated in several diseases and is known to spread in a prion-like manner. There is a relationship between protein aggregate structure (strain) and clinical phenotype in prion diseases, however, whether differences in the strains of α-synuclein aggregates account for the different pathologies remained unclear. Here, we generated two types of α-synuclein fibrils from identical monomer and investigated their seeding and propagation ability in mice and primary-cultured neurons. One α-synuclein fibril induced marked accumulation of phosphorylated α-synuclein and ubiquitinated protein aggregates, while the other did not, indicating the formation of α-synuclein two strains. Notably, the former α-synuclein strain inhibited proteasome activity and co-precipitated with 26S proteasome complex. Further examination indicated that structural differences in the C-terminal region of α-synuclein strains lead to different effects on proteasome activity. These results provide a possible molecular mechanism to account for the different pathologies induced by different α-synuclein strains.
Keywords: mouse; neuroscience; prion; proteasome; strain.
© 2020, Suzuki et al.
Conflict of interest statement
GS, SI, MH, RK, TN, SH, YS, MH No competing interests declared
Figures
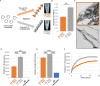
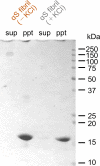
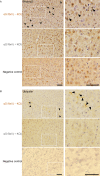
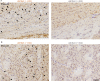
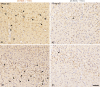
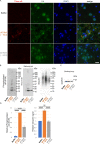
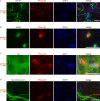
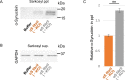
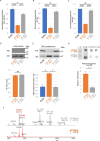
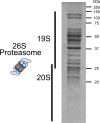
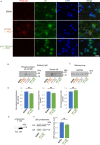
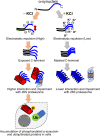
Similar articles
-
α-Synuclein conformational strains spread, seed and target neuronal cells differentially after injection into the olfactory bulb.Acta Neuropathol Commun. 2019 Dec 30;7(1):221. doi: 10.1186/s40478-019-0859-3. Acta Neuropathol Commun. 2019. PMID: 31888771 Free PMC article.
-
Cell-produced alpha-synuclein oligomers are targeted to, and impair, the 26S proteasome.Neurobiol Aging. 2010 Jun;31(6):953-68. doi: 10.1016/j.neurobiolaging.2008.07.008. Epub 2008 Aug 20. Neurobiol Aging. 2010. PMID: 18715677
-
14-3-3 Proteins Reduce Cell-to-Cell Transfer and Propagation of Pathogenic α-Synuclein.J Neurosci. 2018 Sep 19;38(38):8211-8232. doi: 10.1523/JNEUROSCI.1134-18.2018. Epub 2018 Aug 9. J Neurosci. 2018. PMID: 30093536 Free PMC article.
-
Dissecting the potential molecular mechanisms underlying alpha-synuclein cell-to-cell transfer in Parkinson's disease.Parkinsonism Relat Disord. 2009 Dec;15 Suppl 3:S143-7. doi: 10.1016/S1353-8020(09)70802-8. Parkinsonism Relat Disord. 2009. PMID: 20082977 Review.
-
Posttranslational Modifications and Clearing of α-Synuclein Aggregates in Yeast.Biomolecules. 2015 Apr 23;5(2):617-34. doi: 10.3390/biom5020617. Biomolecules. 2015. PMID: 25915624 Free PMC article. Review.
Cited by
-
Novel self-replicating α-synuclein polymorphs that escape ThT monitoring can spontaneously emerge and acutely spread in neurons.Sci Adv. 2020 Oct 2;6(40):eabc4364. doi: 10.1126/sciadv.abc4364. Print 2020 Oct. Sci Adv. 2020. PMID: 33008896 Free PMC article.
-
Quaternary structure of patient-homogenate amplified α-synuclein fibrils modulates seeding of endogenous α-synuclein.Commun Biol. 2022 Sep 30;5(1):1040. doi: 10.1038/s42003-022-03948-y. Commun Biol. 2022. PMID: 36180728 Free PMC article.
-
"Janus-Faced" α-Synuclein: Role in Parkinson's Disease.Front Cell Dev Biol. 2021 May 28;9:673395. doi: 10.3389/fcell.2021.673395. eCollection 2021. Front Cell Dev Biol. 2021. PMID: 34124057 Free PMC article. Review.
-
Alpha-Synuclein Aggregation Pathway in Parkinson's Disease: Current Status and Novel Therapeutic Approaches.Cells. 2022 May 24;11(11):1732. doi: 10.3390/cells11111732. Cells. 2022. PMID: 35681426 Free PMC article. Review.
-
Dissecting aggregation and seeding dynamics of α-Syn polymorphs using the phasor approach to FLIM.Commun Biol. 2022 Dec 8;5(1):1345. doi: 10.1038/s42003-022-04289-6. Commun Biol. 2022. PMID: 36477485 Free PMC article.
References
Publication types
MeSH terms
Substances
Grants and funding
- 16K21650/Japan Society for the Promotion of Science/International
- 26117005/Ministry of Education, Culture, Sports, Science, and Technology/International
- JPMJCR18H3/Core Research for Evolutional Science and Technology/International
- JP18dm0207019/Japan Agency for Medical Research and Development/International
LinkOut - more resources
Full Text Sources