Mapping of the FGF14:Nav1.6 complex interface reveals FLPK as a functionally active peptide modulating excitability
- PMID: 32671946
- PMCID: PMC7363588
- DOI: 10.14814/phy2.14505
Mapping of the FGF14:Nav1.6 complex interface reveals FLPK as a functionally active peptide modulating excitability
Abstract
The voltage-gated sodium (Nav) channel complex is comprised of pore-forming α subunits (Nav1.1-1.9) and accessory regulatory proteins such as the intracellular fibroblast growth factor 14 (FGF14). The cytosolic Nav1.6 C-terminal tail binds directly to FGF14 and this interaction modifies Nav1.6-mediated currents with effects on intrinsic excitability in the brain. Previous studies have identified the FGF14V160 residue within the FGF14 core domain as a hotspot for the FGF14:Nav1.6 complex formation. Here, we used three short amino acid peptides around FGF14V160 to probe for the FGF14 interaction with the Nav1.6 C-terminal tail and to evaluate the activity of the peptide on Nav1.6-mediated currents. In silico docking predicts FLPK to bind to FGF14V160 with the expectation of interfering with the FGF14:Nav1.6 complex formation, a phenotype that was confirmed by the split-luciferase assay (LCA) and surface plasmon resonance (SPR), respectively. Whole-cell patch-clamp electrophysiology studies demonstrate that FLPK is able to prevent previously reported FGF14-dependent phenotypes of Nav1.6 currents, but that its activity requires the FGF14 N-terminal tail, a domain that has been shown to contribute to Nav1.6 inactivation independently from the FGF14 core domain. In medium spiny neurons in the nucleus accumbens, where both FGF14 and Nav1.6 are abundantly expressed, FLPK significantly increased firing frequency by a mechanism consistent with the ability of the tetrapeptide to interfere with Nav1.6 inactivation and potentiate persistent Na+ currents. Taken together, these results indicate that FLPK might serve as a probe for characterizing molecular determinants of neuronal excitability and a peptide scaffold to develop allosteric modulators of Nav channels.
Keywords: accessory protein; excitability; inactivation; sodium channels.
© 2020 The Authors. Physiological Reports published by Wiley Periodicals, Inc. on behalf of The Physiological Society and the American Physiological Society.
Conflict of interest statement
Dr. Fernanda Laezza is a founder and owns stocks in IonTx Inc.
Figures
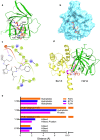
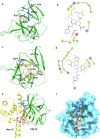
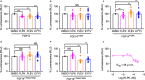
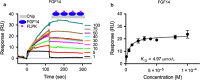
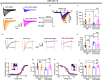
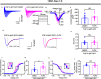
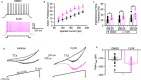
Similar articles
-
Identification of Amino Acid Residues in Fibroblast Growth Factor 14 (FGF14) Required for Structure-Function Interactions with Voltage-gated Sodium Channel Nav1.6.J Biol Chem. 2016 May 20;291(21):11268-84. doi: 10.1074/jbc.M115.703868. Epub 2016 Mar 18. J Biol Chem. 2016. PMID: 26994141 Free PMC article.
-
Identification of peptidomimetics as novel chemical probes modulating fibroblast growth factor 14 (FGF14) and voltage-gated sodium channel 1.6 (Nav1.6) protein-protein interactions.Bioorg Med Chem Lett. 2019 Feb 1;29(3):413-419. doi: 10.1016/j.bmcl.2018.12.031. Epub 2018 Dec 15. Bioorg Med Chem Lett. 2019. PMID: 30587448 Free PMC article.
-
Modulation of the FGF14:FGF14 homodimer interaction through short peptide fragments.CNS Neurol Disord Drug Targets. 2014;13(9):1559-70. doi: 10.2174/1871527313666141126103309. CNS Neurol Disord Drug Targets. 2014. PMID: 25426956 Free PMC article.
-
Bidirectional Modulation of the Voltage-Gated Sodium (Nav1.6) Channel by Rationally Designed Peptidomimetics.Molecules. 2020 Jul 24;25(15):3365. doi: 10.3390/molecules25153365. Molecules. 2020. PMID: 32722255 Free PMC article.
-
Intracellular Fibroblast Growth Factor 14: Emerging Risk Factor for Brain Disorders.Front Cell Neurosci. 2017 Apr 19;11:103. doi: 10.3389/fncel.2017.00103. eCollection 2017. Front Cell Neurosci. 2017. PMID: 28469558 Free PMC article. Review.
Cited by
-
Differential regulation of cardiac sodium channels by intracellular fibroblast growth factors.J Gen Physiol. 2023 May 1;155(5):e202213300. doi: 10.1085/jgp.202213300. Epub 2023 Mar 21. J Gen Physiol. 2023. PMID: 36944081 Free PMC article.
-
Glycogen Synthase Kinase 3: Ion Channels, Plasticity, and Diseases.Int J Mol Sci. 2022 Apr 16;23(8):4413. doi: 10.3390/ijms23084413. Int J Mol Sci. 2022. PMID: 35457230 Free PMC article. Review.
-
Pharmacologically Targeting the Fibroblast Growth Factor 14 Interaction Site on the Voltage-Gated Na+ Channel 1.6 Enables Isoform-Selective Modulation.Int J Mol Sci. 2021 Dec 17;22(24):13541. doi: 10.3390/ijms222413541. Int J Mol Sci. 2021. PMID: 34948337 Free PMC article.
-
Differential Modulation of the Voltage-Gated Na+ Channel 1.6 by Peptides Derived From Fibroblast Growth Factor 14.Front Mol Biosci. 2021 Sep 7;8:742903. doi: 10.3389/fmolb.2021.742903. eCollection 2021. Front Mol Biosci. 2021. PMID: 34557523 Free PMC article.
-
Neurovirulent cytokines increase neuronal excitability in a model of coronavirus-induced neuroinflammation.Intensive Care Med Exp. 2023 Oct 14;11(1):71. doi: 10.1186/s40635-023-00557-9. Intensive Care Med Exp. 2023. PMID: 37833408 Free PMC article.
References
-
- Alshammari, T. K. , Alshammari, M. A. , Nenov, M. N. , Hoxha, E. , Cambiaghi, M. , Marcinno, A. , … Laezza, F. (2016). Genetic deletion of fibroblast growth factor 14 recapitulates phenotypic alterations underlying cognitive impairment associated with schizophrenia. Transl Psychiatry, 6(5), e806–e806 10.1038/tp.2016.66. - DOI - PMC - PubMed
-
- Andrei, S. A. , Sijbesma, E. , Hann, M. , Davis, J. , O'Mathony, G. , Perry, M. W. D. , … Ottmann, C. (2017). Stablization of protein‐protein interaction in drug discovery. Expert Opinion on Drug Discovery, 12(9), 925–940. - PubMed
Publication types
MeSH terms
Substances
Grants and funding
LinkOut - more resources
Full Text Sources
Research Materials