Karrikin Signaling Acts Parallel to and Additively with Strigolactone Signaling to Regulate Rice Mesocotyl Elongation in Darkness
- PMID: 32665307
- PMCID: PMC7474294
- DOI: 10.1105/tpc.20.00123
Karrikin Signaling Acts Parallel to and Additively with Strigolactone Signaling to Regulate Rice Mesocotyl Elongation in Darkness
Abstract
Seedling emergence in monocots depends mainly on mesocotyl elongation, requiring coordination between developmental signals and environmental stimuli. Strigolactones (SLs) and karrikins are butenolide compounds that regulate various developmental processes; both are able to negatively regulate rice (Oryza sativa) mesocotyl elongation in the dark. Here, we report that a karrikin signaling complex, DWARF14-LIKE (D14L)-DWARF3 (D3)-O. sativa SUPPRESSOR OF MAX2 1 (OsSMAX1) mediates the regulation of rice mesocotyl elongation in the dark. We demonstrate that D14L recognizes the karrikin signal and recruits the SCFD3 ubiquitin ligase for the ubiquitination and degradation of OsSMAX1, mirroring the SL-induced and D14- and D3-dependent ubiquitination and degradation of D53. Overexpression of OsSMAX1 promoted mesocotyl elongation in the dark, whereas knockout of OsSMAX1 suppressed the elongated-mesocotyl phenotypes of d14l and d3 OsSMAX1 localizes to the nucleus and interacts with TOPLESS-RELATED PROTEINs, regulating downstream gene expression. Moreover, we showed that the GR24 enantiomers GR245DS and GR24 ent-5DS specifically inhibit mesocotyl elongation and regulate downstream gene expression in a D14- and D14L-dependent manner, respectively. Our work revealed that karrikin and SL signaling play parallel and additive roles in modulating downstream gene expression and negatively regulating mesocotyl elongation in the dark.
© 2020 American Society of Plant Biologists. All rights reserved.
Figures
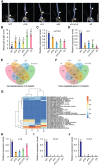
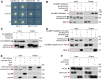
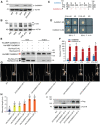

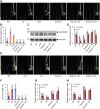
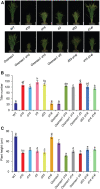
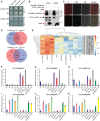
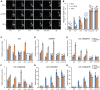
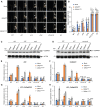
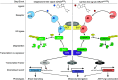
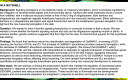
Similar articles
-
Strigolactone and Karrikin Signaling Pathways Elicit Ubiquitination and Proteolysis of SMXL2 to Regulate Hypocotyl Elongation in Arabidopsis.Plant Cell. 2020 Jul;32(7):2251-2270. doi: 10.1105/tpc.20.00140. Epub 2020 Apr 30. Plant Cell. 2020. PMID: 32358074 Free PMC article.
-
Downregulation of rice DWARF 14 LIKE suppress mesocotyl elongation via a strigolactone independent pathway in the dark.J Genet Genomics. 2015 Mar 20;42(3):119-24. doi: 10.1016/j.jgg.2014.12.003. Epub 2015 Jan 9. J Genet Genomics. 2015. PMID: 25819088
-
Signalling and responses to strigolactones and karrikins.Curr Opin Plant Biol. 2014 Oct;21:23-29. doi: 10.1016/j.pbi.2014.06.003. Epub 2014 Jul 1. Curr Opin Plant Biol. 2014. PMID: 24996032 Review.
-
Strigolactones negatively regulate mesocotyl elongation in rice during germination and growth in darkness.Plant Cell Physiol. 2010 Jul;51(7):1136-42. doi: 10.1093/pcp/pcq075. Epub 2010 May 24. Plant Cell Physiol. 2010. PMID: 20498118 Free PMC article.
-
Smoke and Hormone Mirrors: Action and Evolution of Karrikin and Strigolactone Signaling.Trends Genet. 2016 Mar;32(3):176-188. doi: 10.1016/j.tig.2016.01.002. Epub 2016 Feb 2. Trends Genet. 2016. PMID: 26851153 Free PMC article. Review.
Cited by
-
SMAX1 potentiates phytochrome B-mediated hypocotyl thermomorphogenesis.Plant Cell. 2022 Jul 4;34(7):2671-2687. doi: 10.1093/plcell/koac124. Plant Cell. 2022. PMID: 35478037 Free PMC article.
-
Diversification of plant SUPPRESSOR OF MAX2 1 (SMAX1)-like genes and genome-wide identification and characterization of cotton SMXL gene family.BMC Plant Biol. 2023 Sep 11;23(1):419. doi: 10.1186/s12870-023-04421-6. BMC Plant Biol. 2023. PMID: 37691127 Free PMC article.
-
Effects of gibberellin priming on seedling emergence and transcripts involved in mesocotyl elongation in rice under deep direct-seeding conditions.J Zhejiang Univ Sci B. 2021 Dec 15;22(12):1002-1021. doi: 10.1631/jzus.B2100174. J Zhejiang Univ Sci B. 2021. PMID: 34904413 Free PMC article. English.
-
Ethylene Signaling Facilitates Plant Adaption to Physical Barriers.Front Plant Sci. 2021 Jul 29;12:697988. doi: 10.3389/fpls.2021.697988. eCollection 2021. Front Plant Sci. 2021. PMID: 34394151 Free PMC article. Review.
-
KARRIKIN UPREGULATED F-BOX 1 negatively regulates drought tolerance in Arabidopsis.Plant Physiol. 2022 Nov 28;190(4):2671-2687. doi: 10.1093/plphys/kiac336. Plant Physiol. 2022. PMID: 35822606 Free PMC article.
References
-
- Akiyama K., Matsuzaki K., Hayashi H.(2005). Plant sesquiterpenes induce hyphal branching in arbuscular mycorrhizal fungi. Nature 435: 824–827. - PubMed
-
- Al-Babili S., Bouwmeester H.J.(2015). Strigolactones, a novel carotenoid-derived plant hormone. Annu. Rev. Plant Biol. 66: 161–186. - PubMed
-
- Alder A., Jamil M., Marzorati M., Bruno M., Vermathen M., Bigler P., Ghisla S., Bouwmeester H., Beyer P., Al-Babili S.(2012). The path from β-carotene to carlactone, a strigolactone-like plant hormone. Science 335: 1348–1351. - PubMed
-
- Arite T., Iwata H., Ohshima K., Maekawa M., Nakajima M., Kojima M., Sakakibara H., Kyozuka J.(2007). DWARF10, an RMS1/MAX4/DAD1 ortholog, controls lateral bud outgrowth in rice. Plant J. 51: 1019–1029. - PubMed
Publication types
MeSH terms
Substances
LinkOut - more resources
Full Text Sources
Research Materials
Miscellaneous